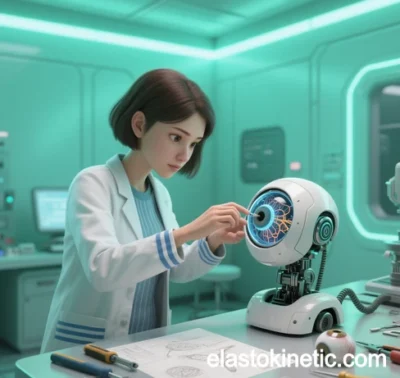
Elastokinetics and the Path to Adaptive Living Machines
Elastokinetics, an interdisciplinary field combining elasticity and dynamics, studies material deformation, energy dissipation, and adaptive response mechanisms under dynamic loads. Its synergy with biological systems—such as muscle contraction, cell migration, and tissue repair—provides critical insights for developing adaptive living machines. Below are key innovations and case studies merging elastokinetic principles with biomaterials science.
I. Elastokinetic-Driven Adaptive Materials
- Stimuli-Responsive Elastin-like Polymers (ELRs)
- Dynamic Structural Reorganization: ELRs, composed of repetitive amino acid sequences (e.g., VPGXG), undergo phase transitions in response to temperature, pH, or ionic strength, forming reversible nanoscale hydrogels. These self-assemble into nanoparticles at body temperature for targeted drug delivery or tissue scaffolding.
- Energy Dissipation and Self-Healing: Entropy-driven elasticity allows ELRs to dissipate energy through molecular chain slippage, avoiding permanent damage. Dynamic covalent bonds (e.g., disulfide bridges) enable self-repair, ideal for flexible robotic joints or artificial vessels.
- Bioinspired Muscle Actuators
- Electroactive Actuators: Piezoelectric polymer-based actuators (e.g., PVDF) generate microcurrents via cerebrospinal fluid flow or external fields, mimicking muscle contraction-relaxation cycles. Self-powered systems can simultaneously release neurotrophic factors and stimulate nerve regeneration.
- Stiffness Modulation: Electrostatic actuators adjust polymer chain alignment in real time, enabling robots to switch between delicate manipulation and high-load tasks.
II. Core Mechanisms of Adaptive Living Machines
- Feedback-Driven Energy-Information Synergy
- Enzyme-Powered Cycles: Enzyme-driven vesicles (e.g., glucose oxidase/catalase systems) create chemical gradients for autonomous motion while regulating microenvironments via metabolic byproducts (e.g., oxygen).
- Metabolic Pathway Integration: Light-driven nanomotors in synthetic cells convert environmental glucose to ATP via glycolysis, enabling sustained motion responsive to fuel availability.
- Mechanochemical Signal Coupling
- Single-Molecule Elasticity: Atomic force microscopy reveals protein conformational elasticity (e.g., fibronectin), guiding the design of biomaterials that transmit mechanical signals. Stiffness-gradient hydrogels direct stem cell differentiation.
- Force-Dependent Molecular Switches: Mechanosensitive peptides (e.g., Talin fragments) in elastokinetic materials expose hidden binding sites under strain, activating intracellular pathways (e.g., YAP/TAZ) to convert mechanical cues into genetic responses.
III. Applications and Case Studies
- Dynamic Drug Delivery Systems
- Tumor Microenvironment Targeting: ELR nanoparticles release chemotherapeutics and generate nitric oxide in high-ROS tumor regions, enhancing permeability via chemodynamic therapy.
- Blood-Brain Barrier Penetration: Magnetic elastomeric nanomotors guided by external fields use ultrasound to transiently open tight junctions, boosting brain drug concentrations.
- Soft Robotics
- Light-Driven Nanomotors: Gold-polymer nanocomposites generate ultrahigh propulsion forces via laser-induced contraction-expansion cycles, enabling microfluidic manipulation or intracellular surgery.
- Adaptive Grippers: ELR-based grippers auto-adjust clamping force using embedded piezoelectric sensors, preventing damage to fragile objects.
- Tissue Engineering and Regeneration
- Dynamic Scaffolds: Porous ELR hydrogels mimic extracellular matrix stiffness changes under cyclic loading, promoting cartilage regeneration.
- Neural Interfaces: Elastic conductive polymers (e.g., PEDOT:PSS-ELR blends) combine flexibility and electroactivity for long-term, high-fidelity neural recording.
IV. Challenges and Future Directions
- Multiscale Mechanical Coupling
- Current models lack precision in cross-scale (molecular-to-tissue) mechanical simulations. Quantum computing and molecular dynamics could refine multiphysics algorithms.
- Energy and Control Systems
- Limited energy density in self-sustaining systems (e.g., <24-hour enzyme-driven operation) requires hybrid light-chemical energy strategies or biofuel cells.
- AI and digital twins could enhance autonomy, such as simulating nanomotor behavior using MRI-derived organ models.
- Ethics and Biosafety
- Autonomous nanosystems need fail-safes (e.g., pH-sensitive self-destruction or light-triggered decomposition) to mitigate irreversible risks.
V. Conclusion
Elastokinetics and biomaterials are converging to create third-generation adaptive living machines with:
- Core Features: Environmental sensing, energy autonomy, dynamic reconfiguration, and collective intelligence.
- Ultimate Goal: Transitioning from preprogrammed machines to lifelike systems, such as evolvable nanoscale communities or self-repairing organ-on-chip platforms.
Future integration with synthetic biology and quantum sensing may enable breakthroughs like cellular-scale “nano-surgeons” or distributed ecological repair systems.
Data sourced from public references. For collaboration or domain inquiries, contact: chuanchuan810@gmail.com