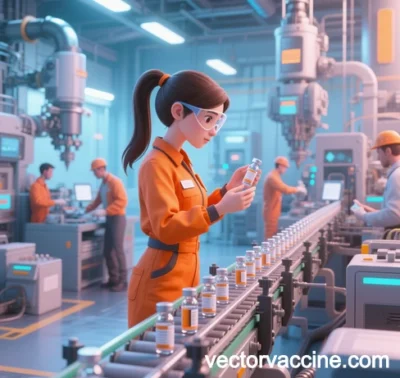
Vector Vaccines: A Cornerstone Tool Against Emerging Infections, Cancer, and Genetic Diseases
Core Mechanisms and Technical Principles
Vector vaccines use engineered viruses or bacteria as delivery vehicles to introduce foreign antigen genes into host cells, triggering specific immune responses. Key principles include:
- Vector Selection and Engineering:
- Viral Vectors: Adenoviruses (AdV), poxviruses (VACV), vesicular stomatitis virus (VSV), and lentiviruses are widely used. For example, adenoviral vectors (e.g., Ad26, ChAdOx1) were pivotal in COVID-19 vaccines (e.g., AstraZeneca) due to high infection efficiency and large gene-carrying capacity.
- Bacterial Vectors: Attenuated Salmonella or Listeria deliver antigens orally to stimulate mucosal immunity.
- Non-Viral Vectors: Lipid nanoparticles or liposomes physically encapsulate nucleic acid antigens.
- Immune Activation:
- Viral vectors infect host cells, translating antigen genes into proteins presented via MHC complexes to activate CD8+ T cells (cellular immunity) and B cells (humoral immunity).
- Bacterial vectors deliver antigens to antigen-presenting cells (APCs), activating Th1/Th2 immune responses.
- Gene Editing and Multifunctionalization:
- CRISPR optimizes vector design (e.g., deleting viral replication genes for safety or inserting multi-antigen genes for broader immunity).
- Lentiviral vectors excel in genetic disease therapy due to non-integrating properties and sustained gene expression.
Applications in Combating Emerging Infections
- Rapid Response to Novel Pathogens:
- COVID-19 Vaccines: Adenoviral vector vaccines (e.g., AstraZeneca’s ChAdOx1-nCoV, J&J’s Ad26.COV2.S) complemented mRNA vaccines, addressing early production shortages and eliminating ultra-cold storage needs.
- Ebola Vaccines: VSV-based Ervebo® and adenoviral Zabdeno® deliver glycoprotein genes, inducing >90% protection via neutralizing antibodies and T-cell responses.
- HIV/Tuberculosis: VSV vectors induce T-cell responses against HIV envelope proteins in preclinical models; adenoviral H56:IC31 is under clinical evaluation for TB prevention.
- Addressing Pathogen Evolution:
- Broad-spectrum antigens (e.g., influenza HA/NA combinations) or CRISPR-designed conserved epitopes counteract viral escape mutations.
- Self-spreading vaccines use engineered vectors to propagate among wildlife, blocking zoonotic transmission (e.g., rabies).
Breakthroughs in Cancer Immunotherapy
- Tumor Antigen-Specific Vaccines:
- Viral vectors express tumor-associated antigens (e.g., MUC1, NY-ESO-1) to activate CD8+ T cells. HPV-targeted vaccines expressing E6/E7 antigens drive immune clearance of cervical cancer.
- Neoantigen vaccines use lentiviral vectors to deliver personalized antigen combinations, advancing in melanoma and lung cancer trials.
- CAR-T Cell Enhancement:
- Lentiviral vectors engineer T cells with chimeric antigen receptors (CARs) targeting CD19 or BCMA, improving blood cancer outcomes.
- CRISPR vectors knock out PD-1 or TCR genes to reduce immunosuppression and boost tumor-killing.
- Oncolytic Virotherapy:
- Engineered poxviruses or adenoviruses selectively infect and lyse tumor cells, releasing antigens and expressing immune stimulants (e.g., GM-CSF) to enhance local immunity.
Innovative Potential in Genetic Disease Therapy
- Gene Replacement:
- AAV vectors deliver functional genes (e.g., CFTR for cystic fibrosis, FIX for hemophilia B), restoring physiological functions in clinical trials.
- Lentiviral vectors edit hematopoietic stem cells (e.g., BCL11A knockout for beta-thalassemia) for long-term efficacy.
- CRISPR Precision Repair:
- Lipid nanoparticles (LNPs) deliver CRISPR-Cas9 to liver cells (e.g., targeting PCSK9 to lower cholesterol) or correct dystrophin mutations in Duchenne muscular dystrophy.
- Epigenetic Regulation:
- dCas9 vectors target disease-related gene promoters, using CRISPRa/i to modulate expression in sickle cell disease or alpha-1 antitrypsin deficiency.
Advantages and Challenges
- Strengths:
- High efficacy with single-dose immunity and multi-antigen delivery.
- Cost-effective production and no cold-chain requirements for select vectors.
- Enhanced safety via replication-deficient or non-integrating designs.
- Challenges:
- Preexisting immunity to common vectors (e.g., Ad5) reduces efficacy; rare serotypes (e.g., Ad26) or non-human vectors (e.g., chimpanzee adenovirus) mitigate this.
- Scalable manufacturing requires addressing contamination risks and vector stability.
- Ethical concerns include environmental spread of self-spreading vaccines and off-target effects in germline editing.
Future Directions
- Next-Gen Vectors:
- Non-human primate adenoviruses (NHPAd) reduce preexisting immunity for cancer vaccines.
- Self-amplifying RNA vectors combine viral delivery with mRNA technology for potent, durable antigen expression.
- Personalized and Combination Therapies:
- Pair neoantigen vaccines with immune checkpoint inhibitors to overcome immunosuppressive microenvironments.
- Integrate vector vaccines with nano-adjuvants (e.g., TLR agonists) for enhanced responses.
- Global Health Equity:
- Promote technology transfer and open patents to enable low-income countries to produce vector vaccines locally.
Conclusion
Vector vaccines have emerged as foundational tools against emerging infections, cancer, and genetic disorders. From rapid COVID-19 vaccine deployment to CAR-T therapy breakthroughs, their versatility continues to expand. While challenges like preexisting immunity and manufacturing complexity persist, innovations in vector engineering, CRISPR integration, and global collaboration promise safer, more precise therapeutic advancements.
Data sourced from publicly available references. For collaborations or domain inquiries, contact: chuanchuan810@gmail.com.