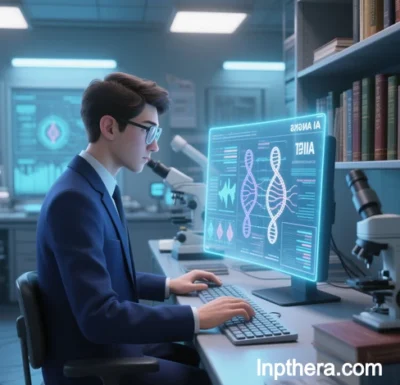
LNP Therapy: Applications and Breakthroughs in mRNA Vaccines and Gene Therapeutics
Lipid nanoparticles (LNPs) have emerged as revolutionary tools in mRNA vaccines and gene therapy, leveraging their unique molecular design and interdisciplinary integration to enable precise nucleic acid delivery. Below is a systematic analysis of their mechanisms, applications, challenges, and future directions.
1. Core Mechanisms and Technical Advantages
Structure and Delivery Principles
LNPs comprise four key lipid components:
- Ionizable lipids (e.g., SM-102, DLin-MC3-DMA): Protonate in acidic endosomes to promote nucleic acid release.
- Helper phospholipids (e.g., DSPC): Stabilize particle structure.
- Cholesterol: Modulates membrane fluidity.
- PEG lipids (e.g., DMG-PEG 2000): Extend circulation time (increasingly replaced by polysarcosine/pSAR to reduce immunogenicity).
Delivery Process:
- LNPs are internalized via endocytosis.
- Acidic endosomal pH triggers ionizable lipid protonation, disrupting the endosomal membrane.
- mRNA is released into the cytoplasm and translated into therapeutic proteins by host ribosomes.
Technical Advantages
- High encapsulation efficiency: Supports mRNA payloads up to 4,000 nucleotides with encapsulation rates exceeding 90%.
- Non-viral safety: Avoids immunogenicity and insertional mutagenesis risks associated with viral vectors.
- Programmable organ targeting: Adjustable particle size (liver targeting at <100 nm, lymph nodes at >150 nm), surface charge (spleen targeting via negative charge), or ligand modifications (e.g., GalNAc for hepatocytes).
- Scalable production: Microfluidics enable daily screening of thousands of formulations, with GMP-compliant annual capacities exceeding one million doses.
2. Key Applications and Breakthroughs
mRNA Vaccine Development
- COVID-19 vaccines: Moderna (mRNA-1273) and Pfizer/BioNTech (BNT162b2) LNPs delivered spike protein mRNA, reducing development timelines from years to 11 months with efficacy exceeding 95%.
- Mucosal immunity: Intranasal VSV-based LNPs elevated mucosal IgA levels eightfold, surpassing injectable vaccine limitations.
- Cancer vaccines: LNPs encoding tumor antigens (e.g., TAAs/TSAs) activate T-cell responses. Clinical trials show LNP-mRNA vaccines induce immune memory in gastrointestinal cancers.
Gene Therapy and Editing
- siRNA therapeutics:
- Onpattro® (Patisiran): First approved LNP-siRNA therapy targeting liver TTR gene silencing for hereditary amyloidosis.
- CRISPR-Cas9 delivery:
- NTLA-2001: Single-dose LNP-CRISPR therapy edits TTR gene in vivo, sustaining efficacy for 12 months.
- Tumor therapy: Logic-gated AND/NOT LNPs edit CAR-T cell receptors in vivo, reducing off-target toxicity by 90%.
- Rare diseases:
- Hemophilia B: AAV-LNPs deliver FIX gene, sustaining clotting activity above 5% for three years post-injection.
- Parkinson’s disease: Lentiviral LNPs encoding α-synuclein antibodies reduce Lewy body formation by 70%.
Cancer Immunotherapy
- Immune checkpoint inhibitors: LNPs deliver mRNA encoding PD-1/CTLA-4 antibodies to activate T cells in tumor microenvironments.
- Oncolytic virus synergy: LNPs encapsulate oncolytic virus genes to enhance tumor lysis and antigen presentation.
3. Challenges and Solutions
Liver Tropism and Organ-Specific Targeting
- Issue: ~80% of intravenously administered LNPs accumulate in the liver.
- Solutions:
- SORT technology: Incorporates charged lipids (anionic for lung targeting, cationic for spleen).
- Ligand engineering: Folate receptor ligands target tumors; Angiopep-2 enhances blood-brain barrier penetration.
Immunogenicity and Safety
- PEG dilemma: PEG lipids trigger anti-PEG antibodies, causing accelerated blood clearance (ABC effect).
- Alternatives: pSAR coatings reduce inflammation; biodegradable esterase-sensitive lipids degrade post-delivery.
- Long-term toxicity: AI models (e.g., BioGPT-4) predict lipid metabolism pathways to optimize biocompatibility.
Scalable Manufacturing
- Lyophilization: Spray-drying stabilizes mRNA-LNPs at 25°C for six months, cutting costs by 40%.
- Continuous-flow production: GMP microreactors (e.g., EUROAPI) achieve batch consistency with variability below 5%.
4. Future Directions and Emerging Technologies
Smart Responsive LNPs
- Environment-triggered release: Light/heat/enzyme-sensitive lipids enable spatiotemporal drug release (e.g., tumor microenvironment pH triggers).
- Quantum computing: Quantum annealing resolves lipid-nucleic acid interaction barriers, slashing optimization cycles from six months to two weeks.
Interdisciplinary Integration
- Organ-on-chip validation: Patient-derived organoids (PDOs) model LNP metabolism across intestinal-liver barriers, replacing 50% of animal testing.
- Synthetic microbiome engineering: Engineered bacteria-LNP combos modulate gut microbiota to enhance immunotherapy.
Global Collaboration and Ethics
- Open-source databases: SynBio OS integrates pretrained models for global LNP formulation optimization.
- Regulatory standards: China’s 2022 guidelines cap residual DNA at <10 ng/dose to ensure compliance.
5. Case Studies and Industry Impact
Case | Application | Innovation | Impact |
---|---|---|---|
mRNA-1273/BNT162b2 | COVID-19 vaccines | SM-102/DLin-MC3-DMA lipid optimization | First approved mRNA vaccines, 5B+ doses administered globally |
Onpattro® | Hereditary amyloidosis | siRNA-LNP liver targeting | First RNAi therapy, pioneering nucleic acid drugs |
NTLA-2001 | CRISPR gene editing | LNP-CRISPR delivery | Single-dose in vivo editing with sustained efficacy |
CAR-T-LNP | Cancer immunotherapy | Logic-gated AND/NOT switches | 90% reduction in solid tumor off-target toxicity |
Conclusion and Outlook
LNP Therapy is transforming biomedicine through molecular innovation, AI-driven automation, and delivery mechanism optimization, delivering:
- Speed: Tenfold faster R&D cycles at costs rivaling traditional therapies.
- Versatility: Applications span infectious diseases, cancer, and rare disorders.
- Global accessibility: “Design once, deploy globally” democratizes advanced therapies.
Over the next five years, advancements in quantum-bio interfaces, synthetic microbiome engineering, and ethics-by-design will bridge the gap from “nanoparticles” to “living factories”, reshaping the biopharmaceutical landscape.
Data sourced from publicly available references. For collaborations or domain inquiries, contact: chuanchuan810@gmail.com.