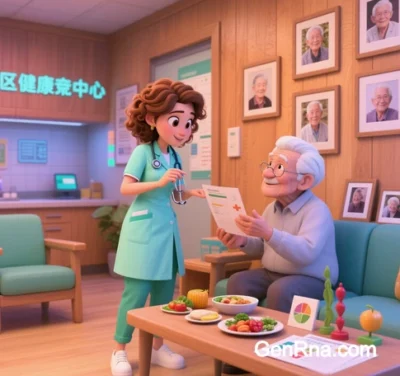
Genome Editing Therapies for Alzheimer’s Disease and Age-Related Disorders: Current Progress and Future Directions
Genome editing technologies, particularly CRISPR-Cas9 systems, have emerged as cutting-edge tools for treating Alzheimer’s disease (AD) and other age-related conditions. By precisely modulating disease-causing genes or repairing mutations, these therapies aim to fundamentally alter disease progression. Below is an in-depth analysis of advancements, clinical outcomes, and challenges in the field.
I. Alzheimer’s Disease: Key Targets and Strategies
1. Amyloid Pathway Intervention
- APP Gene Editing:
CRISPR-Cas9 targets the β-secretase (BACE1) cleavage site or disrupts pathogenic APP variants (e.g., APPswe), significantly reducing β-amyloid (Aβ) production. In transgenic AD mouse models, this approach reduces Aβ plaques and reverses cognitive deficits. - PSEN1 Mutation Repair:
CRISPR-Cas9 selectively disrupts mutant alleles of PSEN1 (e.g., M146L), restoring the Aβ42/40 ratio to normal levels and mitigating neurotoxicity.
2. APOE Gene Regulation
- APOE4 Variant Editing:
APOE4, the strongest genetic risk factor for late-onset AD, is converted into protective APOE3 or modified with the Christchurch mutation (a natural AD-resistant variant) using CRISPR. This reduces tau pathology and neuroinflammation in mouse models. - Epigenetic Silencing:
CRISPR-dCas9 systems suppress APOE4 expression while preserving APOE3 function, validated in humanized mouse models.
3. Tau Pathology Intervention
- MAPT Gene Modification:
Base editing corrects hyperphosphorylation sites (e.g., Ser202/Thr205) on tau protein, reducing neurofibrillary tangles. Duke University’s CRISPR/dCas9 platform successfully lowers phosphorylated tau levels in mice.
4. Neuroprotection and Inflammation Control
- Microglial Reprogramming:
Knocking out pro-inflammatory genes (e.g., TREM2) or activating anti-inflammatory genes (e.g., IL-10) suppresses neuroinflammation and enhances Aβ clearance. MIT-designed CRISPR-Cas9 nanoparticles enable microglia-specific targeting.
II. Advances in Gene Therapies for Other Age-Related Diseases
1. Progeria
- LMNA Gene Repair:
Prime editing directly corrects the LMNA c.1824C>T mutation, reducing nuclear membrane abnormalities in patient fibroblasts and extending lifespan in animal models. - Telomere Extension:
CRISPR activation of telomerase (TERT) or telomeric RNA (TERC) slows telomere attrition in Werner syndrome models.
2. Parkinson’s Disease (PD)
- SNCA Mutation Correction:
Editas Medicine’s base editor repairs the SNCA A53T mutation, restoring dopamine levels to baseline in the substantia nigra. - Mitochondrial DNA Repair:
CRISPR-Cas9 corrects mutations in mitochondrial Complex I genes (e.g., ND4), improving dopaminergic neuron function.
3. Cardiovascular Aging
- Klotho Activation:
Altos Labs’ polygenic editors (KLOTHO, SIRT6) restore vascular elasticity in aged mice and extend healthspan.
III. Technological Breakthroughs and Clinical Challenges
1. Delivery System Innovations
- Blood-Brain Barrier Penetration:
DeepMind’s NeuroLNP, optimized via machine learning, enhances brain delivery efficiency while minimizing liver off-target effects. University of Wisconsin’s silk-derived nanoparticles enable CRISPR-Cas9 transport across the blood-brain barrier. - Spatiotemporal Control:
Optogenetically controlled CRISPR systems (e.g., Opto-CRISPR) enable precise editing in specific brain regions (e.g., hippocampus).
2. Safety Enhancements
- Reduced Off-Target Effects:
High-fidelity Cas9 variants (e.g., HypaCas9) minimize off-target edits, monitored via single-cell sequencing. - Immunogenicity Management:
Stealth viral-like particles (VLPs) evade pre-existing antibodies, and Pfizer’s CleanRibo technology degrades mRNA in non-target cells.
3. Epigenetic Stability
- CRISPR-dCas9/TET1 fusions erase aberrant methylation near editing sites, stabilizing transgene expression.
IV. Ethical and Industrial Challenges
- Germline Editing Limits:
The 2024 Helsinki Declaration Amendment prohibits clinical germline editing but permits in vitro embryo research for progeria gene correction. - Cost and Accessibility:
Treatment costs currently range from 500Kto2M per course. Blockchain-powered microfluidic factories (e.g., Ginkgo BioWorks’ BioFabs) aim to reduce costs to $80,000. - Regulatory Frameworks:
The EU’s NEURA system employs reinforcement learning to balance efficacy and cancer risks during dose optimization.
V. Future Directions and 2030 Outlook
- Multi-Omics Integration:
AI-driven polygenic editing (e.g., co-regulating TERC, SIRT6, NRF2) is projected for clinical use by 2030. - Synthetic Chromosome Engineering:
Artificial chromosome 17p (with telomerase complexes) replaces damaged chromosomes in senescent cells, extending telomeres in primates. - Self-Evolving Editors:
Lentiviral CRISPR-Drive systems adapt therapies in vivo via Monte Carlo Tree Search for multi-stage AD intervention.
Conclusion
Genome editing has transformed AD treatment from delaying pathology to active reversal. Key milestones include:
- 2025: BioViva’s ET-101 (telomerase activation therapy) completing Phase III trials; China’s NMPA approving the epigenetic editor EpiEdit-H3K27 for progeria.
- 2030: Gene editing therapies may extend healthspan by 10–15 years, with a projected market exceeding $24B (Nature Aging).
Challenges remain in delivery precision (e.g., hippocampal coverage), long-term safety (e.g., anti-vector antibodies), and equitable access. Global interdisciplinary collaboration and regulatory harmonization are critical for success.
Data sourced from public references. For collaborations or domain inquiries, contact: chuanchuan810@gmail.com.