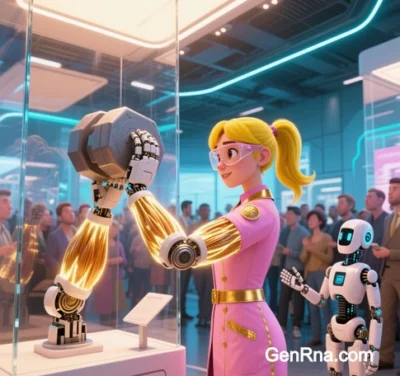
Structure and Function of Artificial Muscles: A Comprehensive Analysis
(As of May 2025)
I. Structural Design: From Biomimicry to Engineering
Artificial muscles are designed to mimic the layered organization and dynamic response mechanisms of biological muscles while surpassing their physical limits. Key structural categories include:
1. Bionic Layered Structures
- Liquid Crystal Elastomer (LCE)-Based Muscles: Utilize reversible molecular realignment under light, heat, or electric fields to achieve strains up to 40%, rivaling natural muscle contraction.
- Carbon Nanotube (CNT) Composites: Woven or helical CNT fibers embedded with conductive/thermoresponsive materials (e.g., paraffin or ionic liquids) enable axial contraction via electrochemical or thermal activation.
- Hydrogel-3D Printed Hybrids: University of Illinois’ biohybrid muscles integrate living cells with hydrogel scaffolds, driven by metabolic activity to replicate “active-passive” biological synergy.
2. Functionalized Drive Units
- Electroactive Polymers (EAPs): Dielectric elastomer films with flexible electrodes deform via Maxwell stress under electric fields, achieving strains exceeding 300%.
- Origami-Inspired Pneumatic Muscles: MIT’s foldable skeletons wrapped in flexible skins achieve programmable shapes (twisting, bending, linear contraction) via vacuum actuation.
3. Energy Transmission and Feedback Systems
- Self-Powered Triboelectric Nanogenerators (TENGs): Convert mechanical motion into electricity for closed-loop control.
- Ionic Polymer-Metal Composites (IPMCs): Ion migration-driven bending actuators for underwater applications.
II. Functional Capabilities: Beyond Biological Limits
Artificial muscles transcend basic contraction-relaxation, offering multimodal actuation and environmental adaptability:
1. Motion and Power
- High Strain and Speed: LCE muscles achieve millisecond-level response times; CNT fibers contract at 30,000 cycles/second.
- Superhuman Load Capacity: Columbia University’s flexible muscles lift 1,000x their weight with 15x the energy density of natural muscle.
2. Environmental Adaptability
- Extreme Temperature Tolerance: LCEs operate from -50°C to 200°C; CNT aerogel muscles withstand 1,500°C.
- Aquatic and Vacuum Compatibility: IPMCs and hydraulic systems enable motor-free motion underwater or in space.
3. Smart Feedback and Control
- Self-Sensing: Embedded flexible sensors (e.g., ISDEA arrays) monitor strain and load, optimizing motion via AI.
- Neuromorphic Control: Spiking neural networks (SNNs) enable sub-5 ms reflex responses.
III. Key Applications and Breakthroughs
Domain | Application | Technology |
---|---|---|
Medical Rehab | SMA-driven exoskeleton gloves (TENG-ISDEA) | 0°–120° finger flexion; 60% energy saving |
Industrial | Multistate robotic arms (CN/CNT films) | 10 kg payload; ±0.05 mm precision |
Space Exploration | Elastic-joint space manipulators | 500 N impact resistance; 45% energy saving |
Military | DARPA bionic exoskeletons | 100 kg payload; 15 km/h mobility |
IV. Challenges and Future Directions
1. Current Bottlenecks
- Standardization: Fragmented protocols hinder cross-platform integration.
- Biocompatibility Risks: Unclear metabolic pathways for liquid metals and graphene.
2. Emerging Innovations
- Biohybrid Systems: ETH Zurich’s living-mechanical hybrid actuators combine LCEs with myoblasts.
- Adaptive Stiffness: Magneto-rheological materials enable 0.1-second stiffness switching (1:1000 ratio) for disaster-response robots.
Conclusion: A Paradigm Shift from Replacement to Enhancement
Artificial muscles are revolutionizing robotics, healthcare, and defense through biomimetic structures (e.g., LCE molecular switching), multiphysics actuation (light-electric-thermal synergy), and intelligent control (SNNs + self-sensing). Evolving from simple motion replacement to integrated systems for environmental interaction (deep-sea exploration), energy autonomy (TENG harvesting), and AI-driven decision-making, they promise to redefine human-machine symbiosis. Over the next decade, breakthroughs in toxicity evaluation (ISO/TC 299) and scalable production (e.g., serum-free processes) will balance ethical governance (e.g., military augmentation) and global accessibility (low-cost solutions), cementing artificial muscles as core components of the cyborg era.
Data sourced from public references. Contact: chuanchuan810@gmail.com.