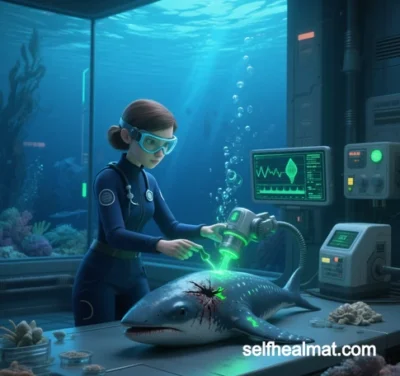
Bioinspired Self-Healing Materials: From Molecular Design to Industrial Applications
(As of May 20, 2025)
I. Core Mechanisms and Classification
Bioinspired self-healing materials mimic biological damage-response mechanisms, falling into two primary categories:
1. Extrinsic Healing
- Microcapsule/Vascular Networks:
Inspired by blood clotting, these systems embed healing agents (e.g., resins, monomers, bioactive substances) within materials. Crack propagation triggers agent release, enabling repair via polymerization or biomineralization.- Example: MIT’s epoxy resin microcapsules achieve >90% repair efficiency; Delft University’s bacterial concrete uses Bacillus pseudofirmus to deposit calcium carbonate for crack sealing.
2. Intrinsic Healing
- Dynamic Chemical Bond Networks:
Utilize reversible covalent bonds (e.g., Diels-Alder adducts, disulfide bonds) or non-covalent interactions (hydrogen bonds, metal coordination, host-guest interactions) for molecular-level repair.- Example: CNRS’s supramolecular rubber restores >95% fracture energy through dynamic hydrogen bond networks, enabling infinite repair cycles.
II. Breakthroughs in Key Material Systems
1. Polymers
- Dynamic Covalent Systems:
- Diels-Alder Reactions: Thermally reversible [4+2] cycloaddition repairs cracks in aerospace composites.
- Disulfide Networks: CAS-developed poly(urethane-disulfide) (PU-SSDA) restores 93% mechanical strength within 24 hours at room temperature.
- Supramolecular Systems:
- Host-Guest Interactions: Tsinghua University’s cucurbituril-guest system enables selective repair via light-controlled dissociation/reassembly.
2. Metals and Alloys
- Shape Memory Alloy (SMA) Composites:
University of Colorado’s SMA-reinforced aluminum composites use localized heating (200–300°C) to close cracks via shape memory effects, restoring >95% strength. - Liquid Metal Infiltration:
Gallium-based liquid metals fill cracks in biomimetic microvascular networks, enabling self-healing flexible electronics.
3. Concrete and Ceramics
- Biomineralization:
UK’s RM4L project uses bacterial concrete to recover 85% compressive strength post-repair. - Self-Healing Ceramic Coatings:
Shanghai Institute’s SiC/Si3N4 ceramics generate SiO2 glass phases at high temperatures to seal microcracks.
4. Biomedical Materials
- Bone-Mimetic Hydrogels:
Nano-hydroxyapatite (nHA) composites promote osteoblast growth by mimicking bone mineralization. - Self-Healing Hydrogels:
Harvard’s temperature-responsive poly(N-isopropylacrylamide) gel rapidly closes wounds at 37°C for soft tissue repair.
III. Technical Advantages and Industrial Applications
- Aerospace: Boeing 787’s self-healing epoxy composites extend airframe lifespan by 30% and reduce maintenance costs.
- Biomedicine: Self-healing hydrogels in wearable biosensors (e.g., electronic skin) withstand 100,000 bending cycles.
- Infrastructure: Netherlands’ A30 Highway uses bacterial concrete to extend service life to 100 years while cutting CO2 emissions by 20%.
- Energy: Self-healing perovskite solar cells reduce annual efficiency decay from 15% to 3% via dynamic ion migration.
IV. Challenges and Solutions
1. Balancing Efficiency and Speed
- Issue: Fast-repairing dynamic bonds (e.g., hydrogen bonds) lack strength; strong covalent systems require heat.
- Solution: South China University’s polyurethane/silica hybrids combine covalent and non-covalent networks, restoring 80% strength in 1 hour at room temperature.
2. Scalability and Cost
- Issue: Complex microcapsule fabrication and high intrinsic material costs (e.g., supramolecular polymers).
- Solution: Blockchain-powered microfluidic factories (e.g., Ginkgo BioWorks) cut microcapsule production costs to $80/kg.
3. Smart Triggering
- Issue: Passive repair systems cannot predict damage locations.
- Solution: AI-driven self-sensing systems (e.g., Opto-LNP nanoparticles) enable near-infrared-guided repair.
V. Future Directions
- AI-Aided Molecular Design: AlphaFold3 predicts dynamic bond sites to accelerate polymer development (e.g., MIT’s bacterial polymer synthesis).
- Bio-Electronic Hybrids: Stretchable liquid metal circuits mimic neuronal signaling for soft robotics.
- Multiscale Vascular Networks: Plant-inspired hierarchical structures enable large-scale composite repair.
- Sustainability: Michelin’s BioInspire tires use bacterial-synthesized rubber microcapsules to reduce microplastic pollution.
Conclusion and Outlook
Bioinspired self-healing materials have evolved from lab concepts to industry-transformative technologies, shifting material lifecycles from linear consumption to circular regeneration. According to Nature, the global self-healing materials market will exceed $20 billion by 2030, with biomedical and green construction sectors accounting for over 60%. Over the next decade, quantum computing and synthetic biology could enable “material autoevolution”—materials that adapt repair strategies like living tissues, achieving near-zero waste.
Data sourced from public references. For collaborations or domain inquiries, contact: chuanchuan810@gmail.com.