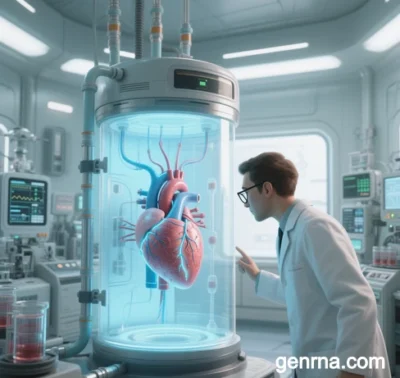
Challenges and Breakthroughs in Artificial Organ Development
Artificial organ technology, a revolutionary field combining life sciences and engineering, aims to create functional replacements for failing human organs through bioengineering, materials science, and AI. Despite rapid advancements, its industrialization faces significant challenges alongside critical breakthroughs. Below is an analysis of technical hurdles and innovations:
I. Core Challenges
- Biological and Fundamental Science Barriers
- Directed Cell Differentiation and Proliferation:
Stem cells possess pluripotency, but efficiently guiding their differentiation into specific functional cells (e.g., cardiomyocytes, hepatocytes) remains unstable and inefficient. Organoid cultures often exhibit uncontrolled heterogeneity and differentiation pathways. - 3D Architecture and Vascularization:
Replicating complex organ structures (e.g., heart chambers, liver lobules) in vitro is difficult. The absence of functional vascular networks leads to hypoxia and limits organ size. - Neural Integration and Functional Synergy:
Post-transplant integration with the host nervous system is limited, resulting in functional isolation (e.g., artificial hearts unresponsive to autonomic regulation).
- Directed Cell Differentiation and Proliferation:
- Material and Engineering Obstacles
- Biomimetic Scaffold Materials:
Current materials (e.g., collagen, polylactic acid) struggle to balance mechanical strength and degradation rates, risking inflammation or structural collapse. - Dynamic Microenvironment Simulation:
In vitro systems fail to replicate biomechanical conditions (e.g., cardiac contractions, pulmonary pressure gradients), causing cellular dysfunction. - Immune Rejection and Biocompatibility:
Non-autologous cells or materials trigger immune attacks, even with immunosuppressants.
- Biomimetic Scaffold Materials:
- Technical Integration and Scalability
- Multi-Cellular Assembly:
Coordinating diverse cell types (epithelial, stromal, immune) into precise spatial arrangements remains unachievable. - Manufacturing Precision and Cost:
3D bioprinting resolution (~50 μm) is insufficient for capillary-level structures, while costs (e.g., $300k/heart) exceed traditional transplants.
- Multi-Cellular Assembly:
- Ethical and Regulatory Constraints
- Embryonic Stem Cell Controversies:
Ethical debates persist, with some countries restricting research. - Gene-Editing Risks:
CRISPR off-target effects and “designer baby” concerns hinder clinical adoption.
- Embryonic Stem Cell Controversies:
II. Technological Breakthroughs
- Stem Cells and Organoid Technology
- Organoid Optimization:
Microfluidic chips simulate nutrient gradients to grow small liver organoids with partial metabolic functions. - Induced Pluripotent Stem Cells (iPSCs):
Autologous iPSC-derived retinal cells show no rejection post-transplant in Japanese trials.
- Organoid Optimization:
- 3D Bioprinting and Biomimetic Design
- High-Precision Bioprinting:
Multi-nozzle systems achieve 10 μm resolution, creating skin tissues with capillary networks. - Dynamic Scaffolds:
Light-responsive hydrogels adjust stiffness to mimic developmental mechanics.
- High-Precision Bioprinting:
- Vascularization and Neural Integration
- Self-Assembling Vasculature:
Gene-edited endothelial cells express VEGF to form functional vascular networks. - Biohybrid Interfaces:
Flexible electronics coupled with neural stem cells enable preliminary electrical coupling in artificial pancreases.
- Self-Assembling Vasculature:
- Gene Editing and Synthetic Biology
- Immune Stealth Design:
CRISPR-mediated α-Gal antigen removal in pig cells reduces xenotransplant rejection by 70%. - Synthetic Biological Circuits:
Engineered cells autonomously secrete insulin in response to glucose levels.
- Immune Stealth Design:
- Interdisciplinary Convergence
- AI-Driven Organ Design:
Deep learning models optimize scaffold architectures for complex liver lobules. - Quantum Biosensing:
Diamond-based probes monitor single-cell mechanical changes to refine organoid cultures.
- AI-Driven Organ Design:
III. Future Pathways
- Modular Organ Assembly:
Build functional units (e.g., nephrons, islets) for robotic assembly into whole organs. - Organ-on-a-Chip Testing:
Sensor-integrated chips replace animal testing for drug screening and toxicity studies. - Engineered Xenotransplants:
CRISPR-edited pig organs (hearts, kidneys) enter Phase II trials to address donor shortages.
IV. Commercialization Challenges
Aspect | Progress | Hurdles |
---|---|---|
Cell Sources | iPSCs reduce ethical risks | High stem cell expansion costs |
Manufacturing | 3D printing efficiency: 1 organ/week | Lack of standardized quality control |
Regulatory Approval | FDA “RMAT” fast-track pathway | EMA requires 10+ years of follow-up |
Market Penetration | Artificial skin market: $420M (2025) | Complex organs (heart, liver) <5% |
Conclusion
Artificial organ technology is transitioning from functional replacement to biological augmentation, driven by advances in stem cells, materials, and AI. While vascularization, neural integration, and scalability remain critical challenges, organoid models, gene editing, and 3D printing are paving the way for industrialization. Over the next decade, interdisciplinary innovation and ethical frameworks could position lab-grown organs as the gold standard for organ failure treatment, reshaping modern medicine.
Data sourced from public references. For collaboration or domain inquiries, contact: chuanchuan810@gmail.com