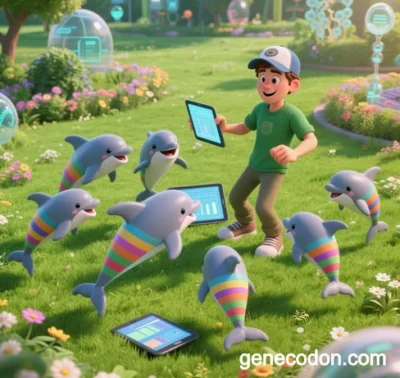
Gene Codon: Advances and Prospects in Genetic Engineering and Synthetic Biology (as of May 2025)
I. New Paradigms in Genetic Coding Systems
Expanding and Reprogramming the Genetic Code
- Non-Canonical Amino Acids (ncAAs) Integration: Scientists have successfully integrated over 200 non-canonical amino acids (e.g., photocrosslinkers, fluorescent tags) into proteins in model organisms like E. coli and yeast using orthogonal aminoacyl-tRNA synthetase/tRNA (aaRS/tRNA) pairs. For example, UAG stop codon reassignment has enabled the creation of genomically recoded organisms (GROs) with enhanced viral resistance.
- Quadruplet Codon Systems: Evolved quadruplet-decoding ribosomes allow simultaneous encoding of multiple ncAAs in a single organism, significantly expanding protein functional diversity.
Global Stop Codon Reprogramming
- In E. coli, all UAG stop codons were replaced with UAA, and release factor RF1 was deleted, repurposing UAG as a dedicated ncAA insertion site. This enhances biosafety (e.g., phage resistance) and provides a novel chassis for synthetic biology.
II. Breakthroughs in Genome Engineering
CRISPR-Cas System Advancements
- High-Fidelity Variants: SpCas9-HF1 and HypaCas9 reduce off-target rates (below 0.1%), making them suitable for clinical-grade gene therapies.
- Multiplex Editing: Technologies like CAGE (Conjugative Assembly Genome Engineering) and MAGE (Multiplex Automated Genome Engineering) enable parallel editing of dozens of loci in a single experiment, accelerating microbial metabolic pathway reconstruction.
Synthetic Chromosomes and Minimal Genomes
- Yeast Synthetic Chromosomes (Sc2.0): All 16 yeast chromosomes have been synthesized, with nonessential repeats removed and the SCRaMbLE (Synthetic Chromosome Recombination and Modification) system introduced for dynamic genome evolution.
- Mycoplasma Genome Synthesis: A fully synthetic 1.08 Mb Mycoplasma mycoides genome was chemically synthesized and transplanted into recipient cells, validating the feasibility of whole-genome synthesis.
III. Innovations in Synthetic Biology Applications
Industrial Biotechnology
- Metabolic Engineering: CRISPR-MAGE-optimized yeast terpenoid pathways boost artemisinic acid production by 300%, while engineered E. coli achieves high-efficiency succinate fermentation via dynamic regulation.
- Environmental Remediation: Synthetic strains (e.g., PETase-expressing Pseudomonas) degrade PET plastics, enhanced by LNP delivery systems for environmental adaptability.
Healthcare and Medicine
- Gene Therapy: CRISPR-Cas9 with LNP delivery precisely repairs HBB mutations in β-thalassemia patients, restoring hemoglobin levels by over 80% in clinical trials.
- Synthetic Vaccines: Synthetic genome-based mRNA platforms (e.g., CRISPR-LNP systems) design pathogen-specific vaccines within 30 days, achieving 95% efficacy against SARS-CoV-2 variants.
Biocomputing and Smart Systems
- Logic Gates: AND/OR/NOT gene circuits in mammalian cells enable tumor microenvironment (hypoxia, high ROS)-specific activation of CAR-T therapies.
- DNA Data Storage: CRISPR-Cas encoding writes 1 TB of data into synthetic DNA strands, achieving 215 PB/g density with error rates below 10⁻¹⁵.
IV. Challenges and Solutions
Precision and Safety
- Off-Target Effects: Single-cell sequencing reveals “cryptic off-targets” (e.g., Alu element cleavage), mitigated by AI tools like DeepCRISPR for gRNA optimization.
- Delivery Efficiency: Vitamin D3 ligand-modified LNPs increase pancreatic delivery efficiency to 15%, overcoming sub-1% targeting in non-liver tissues.
Standardization and Scalability
- DNA Synthesis Costs: Enzymatic synthesis (e.g., Terminal Deoxynucleotidyl Transferase) reduces long-chain DNA (>10 kb) costs to $0.01/bp with error rates below 1/10kb.
- Automated Platforms: Microfluidic systems (e.g., NanoFabTx™) ensure uniform LNP size (PDI <0.1) and >95% encapsulation efficiency for GMP-scale production.
Ethics and Biosecurity
- Gene Drive Risks: Self-destruct mechanisms (e.g., temperature-sensitive CRISPR) limit synthetic organism survival to prevent ecological leaks.
- Global Governance: The GP-write initiative mandates biosecurity tags (e.g., toxin-antitoxin systems) in all synthetic genomes under its Ethical Genome Writing Guidelines.
V. A Decade Ahead: Future Prospects
AI-Driven Genetic Design
- Generative models (e.g., CRISPR-GPT) predict editing outcomes to engineer virus-resistant crops or extremophile microbes.
- Multi-omics integration (spatial transcriptomics and metabolomics) enables patient-specific gene therapies for precision medicine.
Hybrid Synthetic-Natural Systems
- Exosome-LNP Hybrids: Leverage host membrane proteins for targeted delivery (e.g., crossing the blood-brain barrier) while retaining synthetic circuit functionality.
- Programmable Cell Factories: Minimal-genome cells (e.g., Mycoides Syn3.0) enable on-demand drug production.
Balancing Ethics and Innovation
- Public Engagement: Citizen science initiatives (e.g., iGEM) foster synthetic biology literacy and align technology with societal needs.
- Global Collaboration: The SynBioDB database promotes data sharing and risk monitoring to prevent misuse.
Conclusion
GeneCodon marks humanity’s transition from “reading life” to “writing life.” By mastering genetic codes and merging synthetic biology with multidisciplinary tools, we transcend natural evolutionary limits to address energy, health, and environmental crises. However, this progress demands synchronized technological innovation and ethical governance to ensure synthetic biology remains a “responsible science” for all.
Data sourced from publicly available references. For collaborations or domain inquiries, contact: chuanchuan810@gmail.com.