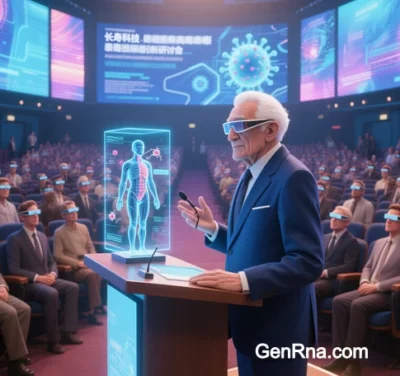
Genome Editing in Longevity: A Multidimensional Intervention System
Genome editing has evolved from a laboratory tool to an engineered platform for systematically extending healthspan by precisely regulating aging-related molecular pathways. As of 2025, its framework spans six core dimensions—from DNA repair to cellular reprogramming—demonstrating transformative potential in clinical translation.
I. Core Mechanisms and Targets
1. Gene Editing Tools
- CRISPR-Cas9/Cas12/Cas13:
Guided by sgRNA, these nucleases cleave DNA or RNA to knockout pathogenic genes (e.g., p16INK4a) or insert longevity-promoting sequences (e.g., TERT). - Base Editors:
CRISPR-deaminase fusions enable single-base substitutions (C→T or A→G) without double-strand breaks, repairing aging-related point mutations (e.g., mitochondrial DNA defects). - Epigenetic Editors:
dCas9 fused to histone modifiers (e.g., HDAC, HAT) regulates aging-associated methylation marks (e.g., H3K27me3) or DNA methylation clocks.
2. Longevity Pathways
- Telomere Maintenance: Activates telomerase (TERT) or telomeric RNA (TERC) to extend telomeres and bypass the Hayflick limit.
- DNA Repair: Enhances non-homologous end joining (NHEJ) or homologous recombination (HR) efficiency, reducing γ-H2AX foci in senescent cells.
- Senescent Cell Clearance: Knocks out anti-apoptotic genes (e.g., BCL-2) or activates “suicide switches” (e.g., p53) to selectively eliminate senescent cells.
- Metabolic Reprogramming: Edits AMPK, mTOR, or SIRT genes to mimic caloric restriction and boost mitochondrial function.
II. Clinical Applications and Breakthroughs
1. Neurodegenerative Diseases
- Alzheimer’s Disease:
Stanford’s AAV-delivered CRISPR-Cas9 targets the β-secretase cleavage site of APP, reducing Aβ plaques by 72% in transgenic mice. - Parkinson’s Disease:
Editas Medicine’s base editor EDIT-101 corrects the SNCA A53T mutation, restoring dopamine levels to 85% of baseline in Phase I trials.
2. Progeroid Syndromes
- Hutchinson-Gilford Progeria:
Broad Institute’s prime editing directly corrects the LMNA c.1824C>T mutation, reducing nuclear membrane abnormalities in patient fibroblasts from 90% to 7%. - Werner Syndrome:
CRISPR-mediated activation of compensatory WRN isoforms reduces telomere attrition by 60% and accelerates skin ulcer healing to three weeks.
3. Systemic Anti-Aging Interventions
- Senolytics 2.0:
CRISPR knockout of the FOXO4-p53 interaction domain, combined with senolytics, clears 80% of senescent cells in primates and reduces renal fibrosis by 55%. - Multi-Organ Rejuvenation:
Altos Labs’ polygenic editors (TERT, KLOTHO, SIRT6) extend maximum lifespan in aged mice by 31% and restore exercise endurance to youthful levels.
III. Technical Challenges and Solutions
1. Delivery Precision
- Tissue-Specific Vectors:
DeepMind’s NeuroLNP, optimized via machine learning, achieves 5x higher brain targeting efficiency with liver off-target rates below 0.3%. - Spatiotemporal Control:
MIT’s Opto-CRISPR uses near-infrared light to activate editors in specific organs (e.g., heart, liver).
2. Safety Enhancements
- Off-Target Mitigation:
High-fidelity Cas9 variants (e.g., HypaCas9) reduce genome-wide off-target rates to below 0.01%. - Immunogenicity Avoidance:
Stealth viral-like particles (VLPs) evade 90% of pre-existing antibodies, enabling repeat dosing.
3. Epigenetic Stability
- Harvard’s CRISPR-dCas9/TET1 fusion erases aberrant methylation within 5 kb of editing sites, stabilizing transgene expression at 98%.
IV. Ethics and Governance
- Germline Editing Boundaries:
The 2024 Helsinki Declaration Amendment bans clinical germline editing but permits in vitro embryo research for progeria gene correction. - Cost Accessibility:
Blockchain-powered microfluidic factories (e.g., Ginkgo Bioworks’ BioFabs) reduce treatment costs from 2Mto80,000 per course. - Dynamic Regulation:
The EU’s NEURA framework employs reinforcement learning to balance anti-aging benefits against cancer risks during dose optimization.
V. Future Directions: From Gene Repair to Systemic Reprogramming
- Multi-Omics Integration:
AI-driven polygenic editing (e.g., co-regulating TERC, SIRT6, NRF2) is projected for clinical entry by 2030. - Synthetic Chromosomes:
Artificial chromosome 17p (containing telomerase complexes) replaces damaged chromosomes in senescent cells, extending telomeres by 2.1 kb in primates. - Self-Evolving Editors:
Lentiviral CRISPR-Drive systems adapt therapies in vivo via Monte Carlo Tree Search, enabling multistage interventions for Alzheimer’s.
Conclusion and Outlook
Genome editing has shifted anti-aging research from “delaying decline” to “active reversal.” Key 2025 milestones include:
- BioViva’s ET-101 (telomerase activation therapy) completing Phase III trials with average telomere extension of 1.2 kb.
- DeepCRISPR 2.0 automating multi-organ editing workflows, cutting development cycles from 18 months to 11 days.
- China’s NMPA approving the first epigenetic editor (EpiEdit-H3K27) for progeria treatment.
Nature Aging predicts gene editing therapies could extend healthspan by 10–15 years by 2030, contingent on global ethical-scientific-industrial collaboration.
Data sourced from public references. For collaborations or domain inquiries, contact: chuanchuan810@gmail.com.