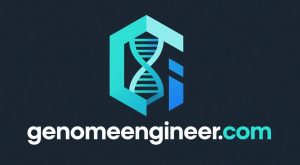
Genome Engineer: An In-Depth Exploration
Genome Engineer refers to the specialized field of systematically designing, editing, or reconstructing the genomes of organisms using biotechnology. Its core objective is to achieve precise manipulation and functional optimization of genomes, integrating the concepts of “genome” and “engineering” to emphasize a rational, design-driven approach to genetic modification. Below is a detailed analysis of its definition, technical systems, applications, and ethical considerations:
I. Definition and Scope
- Core Definition:
Broadly, Genome Engineer encompasses all technologies and practices involving artificial genome intervention, including gene editing, chromosomal engineering, and synthetic genomics. Narrowly, it focuses on targeted genome modification using tools like CRISPR/Cas9, TALENs, and zinc finger nucleases (ZFNs). - Comparison with Traditional Genetic Engineering:FeatureTraditional Genetic EngineeringGenome EngineeringOperational ScaleSingle-gene or short-fragment manipulationMulti-gene, chromosomal, or genome-wide designToolsRecombinant DNA, plasmid vectorsCRISPR-Cas, base editing, prime editingDesign PhilosophyTrial-and-error drivenRational design with high-throughput screeningApplicationsTransgenic organism developmentPrecision medicine, artificial life creationExample: Traditional methods insert foreign insect-resistant genes into crops, while genome engineering optimizes photosynthetic gene networks systemically.
II. Technical Frameworks and Core Tools
- Gene Editing Technologies:
- CRISPR-Cas Systems: Use guide RNA (gRNA) for targeting and Cas nucleases for DNA cleavage, enabling knockouts, insertions, or base substitutions. Derivatives include:
- Base Editing: Converts C→T or A→G without double-strand breaks.
- Prime Editing: Achieves precise point mutations and small insertions via reverse transcriptase.
- TALENs and ZFNs: Protein-DNA recognition modules for targeting high-GC regions or CRISPR-incompatible contexts.
- Synthetic Genomics:
- Whole-Genome Synthesis: Chemically synthesizes minimal functional genomes (e.g., Mycoplasma JCVI-syn3.0) to identify essential genes.
- Chromosomal Engineering: Designs artificial chromosomes (e.g., yeast artificial chromosomes, YACs) for complex metabolic pathway assembly.
- High-Throughput Screening and AI-Assisted Design:
- Multiplex Editing: Edits dozens of loci simultaneously to accelerate functional genomics.
- Deep Learning Models: Predict editing efficiency, off-target effects, and genotype-phenotype relationships to optimize strategies.
III. Applications and Case Studies
- Medicine:
- Gene Therapy: Corrects genetic mutations (e.g., HBB gene in sickle cell anemia) or engineers CAR-T cells with tumor-targeting receptors.
- Pathogen Control: Designs gene drives to suppress malaria mosquito populations or edits viral genomes to reduce virulence.
- Agriculture and Industry:
- Crop Improvement: Edits rice OsSWEET13 for bacterial blight resistance or redesigns microbial metabolic networks for biofuel production.
- Synthetic Biology: Creates “streamlined” industrial strains (e.g., E. coli with non-essential genes removed) to enhance product purity.
- Basic Research:
- Functional Genomics: Genome-wide saturation mutagenesis to map gene networks.
- Evolutionary Studies: Reconstructs ancient genes (e.g., mammoth hemoglobin) to explore adaptive evolution.
IV. Challenges and Ethical Debates
- Technical Limitations:
- Off-Target Effects: Even high-fidelity Cas9 variants (e.g., HiFi-Cas9) carry low-risk unintended edits, potentially causing carcinogenesis.
- Delivery Efficiency: In vivo editing faces challenges with vector capacity (e.g., AAV) and immunogenicity.
- Ethical and Governance Issues:
- Germline Editing: The 2018 “CRISPR babies” incident sparked global condemnation of heritable genome edits.
- Ecological Risks: Gene-drive organisms may disrupt ecosystems, requiring rigorous containment assessments.
- Intellectual Property: CRISPR patent disputes (Broad Institute vs. UC Berkeley) highlight monopolization concerns.
- Global Regulatory Frameworks:
- Divergent Policies: The EU regulates some genome-edited crops as GMOs, while the U.S. and Japan exempt edits without foreign DNA.
- International Collaboration: WHO advocates for a global registry to track clinical trials and environmental releases.
V. Future Directions
- Ultra-Precise Editing:
- Epigenome Editing: Regulates gene expression without altering DNA (e.g., CRISPR-dCas9 fused with demethylases).
- Mitochondrial Genome Editing: Develops CRISPR systems targeting mitochondria to treat maternal genetic diseases.
- Automation and Scalability:
- Robotic Platforms: Integrate AI design, automated pipetting, and phenotypic analysis for closed-loop workflows.
- Cloud Labs: Enables remote submission of editing designs to receive engineered strains within 48 hours.
- Interdisciplinary Integration:
- Quantum Biocomputing: Simulates genome-wide editing outcomes to predict polygenic synergies.
- Neurogenomics: Edits neuronal genomes to study memory encoding and brain-machine interface compatibility.
Conclusion
Genome Engineer represents a paradigm shift from “gene reading/writing” to “genome design,” transforming biological challenges into programmable engineering tasks. Despite hurdles in precision, ethics, and biosafety, its potential in disease treatment, food security, and sustainability is undeniable. As synthetic biology, AI, and automation converge, genome engineering will transition from labs to industries, redefining humanity’s understanding and control of life systems.
Content generated by AI. For inquiries, please contact: chuanchuan810@gmail.com
Genomeengineer(基因组工程师) 是指利用 基因组工程技术(如 CRISPR-Cas9、TALENs 等)对生物体的 DNA 进行精确编辑或改造的专业人员或技术过程。其核心特点如下:
1. 技术范畴
基因编辑:通过工具(如 CRISPR)靶向修改特定基因序列,实现功能增强、修复或沉默。
应用领域:包括疾病治疗(如抗艾滋病基因编辑婴儿1)、农业育种及基础研究。
2. 关键概念
通用性:基因编辑技术(如 CRISPR)在动物、植物、微生物中均可应用,说明基因操作工具的跨物种通用性。
风险:可能因脱靶效应破坏非目标基因,导致不可预见的遗传或健康问题1。
3. 相关术语对比
术语 定义
Genomeengineering 广义的基因组工程化技术,涵盖设计、编辑和合成基因组。
Gene engineering 狭义基因操作,如外源基因插入或修饰(如质粒构建)。