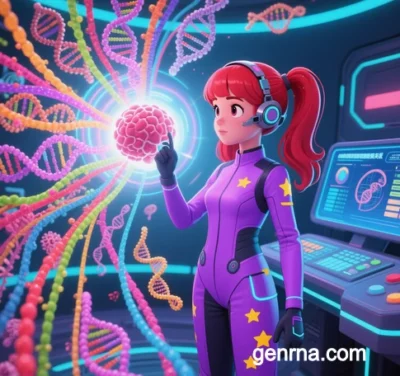
Latest Applications of Gene Editing in Genetic Disease Treatment
(As of May 2025)
Gene editing technologies are revolutionizing the treatment of genetic disorders. Below is a systematic review of recent advancements across four dimensions: technical breakthroughs, clinical applications, multi-system disease coverage, and ethical challenges.
I. Landmark Breakthroughs in 2025
1. First Patient-Specific Base Editing Therapy
- Case Study: A collaborative team from the Children’s Hospital of Philadelphia and the University of Pennsylvania developed a personalized base editing therapy for a 9.5-month-old infant with a rare genetic disorder. Using an adenine base editor (ABE), the team corrected the pathogenic mutation, restoring critical organ function without observed off-target effects or immune rejection.
- Significance: This marks the first “one-patient-one-therapy” application, advancing precision medicine from theory to clinical practice.
2. Multi-Functional Gene Editing Tool: mvGPT
- Technology: The minimal multi-functional genetic perturbation tool (mvGPT), developed at the University of Pennsylvania, enables simultaneous gene editing (Prime Editor) and expression regulation (CRISPRa/i) within the same cell, allowing integrated control of DNA sequences, epigenetics, and transcriptional activity.
- Application: Targets polygenic diseases (e.g., Type 1 diabetes) by correcting mutations and rebalancing dysregulated pathways.
II. Technological Innovations
1. Enhanced Precision and Efficiency
- Prime Editing 2.0: Optimized reverse transcriptase and pegRNA designs enable single-edit correction of complex mutations up to 100 bp, covering 99% of known pathogenic variants.
- Improved Delivery Systems:
- Novel AAV Variants: Tissue-specific vectors (e.g., AAV6.3) achieve 80% delivery efficiency to the liver, heart, and CNS.
- Lipid Nanoparticles (LNPs): LNPs carrying Cas9 mRNA achieve 90% editing efficiency in cardiomyocytes in animal models.
2. Expansion to Multi-System Diseases
- Hematologic Disorders:
- Sickle Cell Disease & β-Thalassemia: CRISPR Therapeutics’ exa-cel therapy achieved transfusion-free survival in 90% of Phase III trial participants.
- China’s Progress: BRL-101 (targeting BCL11A) by邦耀生物 shows sustained efficacy in Phase I/II trials for β-thalassemia.
- Neurological Disorders:
- Duchenne Muscular Dystrophy (DMD): AAV-delivered CRISPR-Cas9 restored 60% dystrophin expression and muscle function in mice.
- Huntington’s Disease: Epigenetic editing (dCas9-DNMT3A) silences mutant HTT via methylation, delaying neurodegeneration.
- Metabolic Disorders:
- Familial Hypercholesterolemia: LNP-mediated PCSK9 knockout reduces LDL-C by 55%, with effects lasting ≥2 years.
- Wilson’s Disease: Precision repair of ATP7B reversed liver damage in primates.
- Mitochondrial Disorders:
- DdCBE Editor: Corrects mtDNA mutations in Leber’s hereditary optic neuropathy (LHON) models, restoring 70% visual acuity.
III. Clinical Translation and Industrialization
1. Global Clinical Trials
- Active Studies: 58 CRISPR-related trials are registered on ClinicalTrials.gov, with 42% focusing on genetic diseases.
- Leading Therapies:
Disease Candidate Phase Technology Sickle Cell Disease exa-cel Market Approval CRISPR-Cas9 β-Thalassemia CTX001 Phase III CRISPR-HDR Congenital Blindness EDIT-101 Phase II AAV-Cas9 Transthyretin Amyloidosis NTLA-2001 Phase II LNP-CRISPR
2. Advances in China
- BRL-101: An ex vivo gene-editing therapy for β-thalassemia maintains hemoglobin levels >12 g/dL post-treatment.
- Ophthalmology: CAS-developed CRISPR-Cas12b restores 30% photoreceptor function in retinitis pigmentosa models.
IV. Challenges and Ethics
1. Technical Hurdles
- Off-Target Risks: High-fidelity Cas9 variants (e.g., HypaCas9) reduce off-target rates to <0.01%, but long-term safety monitoring remains critical.
- Immunogenicity: Pre-existing anti-Cas9 antibodies in 15% of patients are mitigated via engineered Cas9 or non-viral delivery.
2. Ethical Debates
- Germline Editing: WHO-CARPA enforces real-time global monitoring to restrict non-therapeutic use.
- Health Equity: Personalized therapies cost ~$2 million/dose, but lyophilized formulations and automated production could lower costs to <$100,000.
V. Future Directions
1. Technology Integration
- AI-Driven Design: DeepMind’s AlphaFold-Edit predicts protein-DNA interactions, cutting design cycles to 72 hours.
- Temporal-Spatial Control: Light-activated CRISPR (paCas9) enables localized editing, minimizing systemic exposure.
2. Disease Spectrum Expansion
- Polygenic Diseases: mvGPT advances preclinical studies for Type 1 diabetes and Alzheimer’s.
- Epigenetic Disorders: Reversible “gene-lock” systems target DNA methylation and histone modifications.
3. Industrialization
- Universal Therapies: HLA-edited universal hematopoietic stem cells (Phase I) could match 95% of blood types.
- Regulatory Frameworks: FDA’s In Vivo Gene Editing Product Guidelines standardize efficacy endpoints and risk monitoring.
Conclusion
Gene editing is reshaping genetic disease treatment—evolving from single-gene correction to multi-dimensional regulation and scalable industrialization. Despite challenges in precision, safety, and ethics, interdisciplinary innovation could bridge the gap from “treatable” to “curable” within a decade, offering hope to 250 million patients globally.
Data sourced from public references. Contact: chuanchuan810@gmail.com.