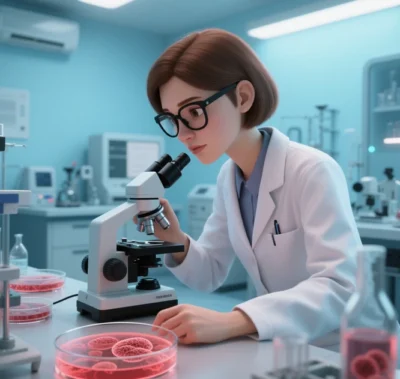
Lipid Nanoparticles (LNPs) in Gene Therapy and Cancer Treatment: Practical Applications
Lipid nanoparticles (LNPs), as non-viral delivery vehicles, have become a core technology in gene therapy and cancer treatment due to their high nucleic acid delivery efficiency, low immunogenicity, and engineering flexibility. Below, we analyze their mechanisms, clinical applications, challenges, and future directions.
I. Technical Mechanisms and Core Advantages
- Structure and Delivery Principles
LNPs are composed of ionizable lipids, phospholipids, cholesterol, and polyethylene glycol (PEG), forming 80–200 nm particles. Their delivery mechanisms rely on:- pH-Responsive Ionizable Lipids: Neutral at physiological pH to minimize blood interactions; positively charged in endosomal acidic environments, promoting membrane fusion and nucleic acid release.
- Enhanced Permeability and Retention (EPR) Effect: Tumor vasculature hyperpermeability and poor lymphatic drainage enable selective LNP accumulation in tumors.
- Key Advantages
- Efficient Nucleic Acid Delivery: Encapsulates mRNA, siRNA, CRISPR systems, and more, protecting them from degradation.
- Targeted Delivery: Surface modifications (e.g., antibodies, aptamers) enable tissue- or cell-specific delivery.
- Low Toxicity: Reduced cytotoxicity and immune activation compared to cationic liposomes.
II. Applications in Gene Therapy
- Nucleic Acid Drug Delivery
- mRNA Therapy:
- Rare Diseases: Moderna’s mRNA-3927 delivers propionyl-CoA carboxylase to hepatocytes via LNPs to restore enzyme activity in propionic acidemia (Phase I/II trials).
- Gene Editing Tools: Co-delivery of CRISPR-Cas9 mRNA and sgRNA enables transient editing with reduced off-target risks (e.g., Intellia’s NTLA-2001 for transthyretin amyloidosis).
- siRNA Therapy: LNPs deliver siRNA to silence oncogenes (e.g., HER2 in breast cancer) or inhibit immune checkpoints (e.g., PD-1).
- Gene-Immunotherapy
- In Vivo CAR-T Cell Engineering: LNPs deliver mRNA-encoded CAR receptors to reprogram T cells directly in patients, bypassing ex vivo expansion (e.g., CD19-targeted CAR-T in leukemia models).
- Immune Cell Editing: Targeted LNP delivery of gene-editing tools to NK cells or macrophages enhances antitumor activity.
III. Applications in Cancer Therapy
- Chemotherapy Delivery
- Liposomal Chemotherapy: Doxil® (doxorubicin liposomes) leverages the EPR effect to increase tumor drug concentration while reducing cardiotoxicity (used in ovarian cancer and Kaposi’s sarcoma).
- Combination Therapy: LNPs co-loaded with chemotherapeutics and immune modulators (e.g., IL-12 mRNA) achieve chemo-immunotherapy synergy.
- Cancer Vaccine Development
- Neoantigen Vaccines: BioNTech’s mRNA-4157 encodes patient-specific neoantigens, activating CD8+ T cells. Combined with PD-1 inhibitors, it improves 2-year survival to 62% in melanoma patients.
- Universal Vaccines: mRNA-LNP vaccines targeting telomerase (hTERT) or carcinoembryonic antigen (CEA) induce broad-spectrum antitumor immunity.
- Tumor Microenvironment Modulation
- Pro-Inflammatory Cytokines: Intratumoral injection of mRNA-LNPs encoding IL-12 or IFN-α reverses immunosuppressive microenvironments.
- Metabolic Pathway Targeting: LNPs deliver siRNA to inhibit tumor glycolysis or fatty acid synthesis (e.g., HK2 or ACC gene silencing).
IV. Targeting Strategies and Innovations
- Active Targeting
- Antibody/Aptamer Conjugation: Anti-EGFR antibody-modified LNPs achieve 82% PLK1 editing efficiency in ovarian cancer cells, significantly extending mouse survival.
- Ligand-Guided Delivery: Folate receptor-targeted LNPs enhance drug accumulation in lung tumors.
- Stimuli-Responsive Designs
- pH/Enzyme-Sensitive Lipids: Release payloads in tumor microenvironments, sparing healthy tissues.
- Photothermal Control: Near-infrared light triggers LNP drug release for spatiotemporal precision.
V. Challenges and Future Directions
- Technical Hurdles
- Delivery Efficiency: Only 10–20% of LNPs reach tumors due to hepatic first-pass effects; lung/brain-targeted carriers (e.g., anti-ACE2 nanoparticles) are needed.
- Long-Term Safety: PEG components may cause allergic reactions; biodegradable lipids are under development.
- Industrialization Barriers
- Cost and Scalability: TdT enzyme-driven DNA synthesis lowers costs, while microfluidic factories reduce production cycles to two weeks.
- Cold Chain Dependence: Lyophilized formulations (e.g., CureVac’s CVnCoV) enable 2–8°C storage, aiding resource-limited regions.
- Future Trends
- AI-Driven LNP Design: Machine learning predicts lipid combinations and in vivo behavior for rational design.
- Multifunctional Platforms: LNPs integrating diagnostics (e.g., fluorescent tags) and therapeutics enable theranostic applications.
VI. Conclusion
LNPs have transitioned from concept to clinical reality, demonstrating transformative potential in gene therapy and cancer treatment. With continued advancements in targeting strategies, manufacturing, and regulatory frameworks, LNPs are poised to become central tools in personalized medicine, bridging the gap from broad-spectrum treatment to precision cures.
Data sourced from public references. For collaboration or domain inquiries, contact: chuanchuan810@gmail.com