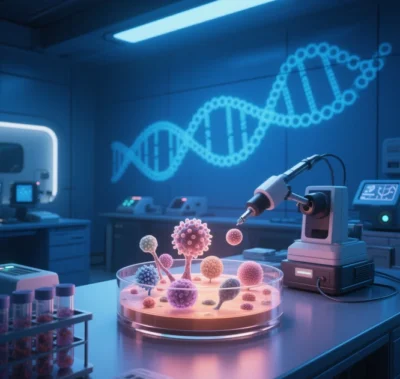
Synthetic Biology (SynBio) and Cell/Gene Therapy: Technological Revolution and Clinical Applications
Synthetic Biology (SynBio) is transforming cell and gene therapy (CGT) through the engineering of biological systems. By integrating biology and engineering, SynBio enables modular, programmable redesign of cellular and genetic functions for precision medicine. Below is a systematic analysis of key breakthroughs, clinical applications, challenges, and future directions.
1. SynBio-Driven Technological Breakthroughs in CGT
Gene Editing Advancements
- CRISPR-Cas Optimization:
Enhanced precision of CRISPR systems (e.g., high-fidelity Cas9, base/prime editing) reduces off-target effects. For example, CRISPR-Cas9 has been used to repair HBB gene mutations in sickle cell anemia. - Multifunctional Editing Platforms:
dCas9 fused with transcriptional regulators (e.g., SRSF1) reprograms splicing to correct disease-related anomalies (e.g., SMN2 in spinal muscular atrophy).
Gene Circuits and Logic Gates
- Dynamic Regulation Systems:
Synthetic gene circuits (e.g., AND/NOT gates) enable environment-responsive therapeutic cells. For instance, AND-gate CAR-T cells activate only in the presence of dual antigens (e.g., CD19+CD22), sparing healthy tissues. - Safety Controls:
Inducible “suicide switches” (e.g., iCas9) eliminate engineered cells during therapy escalation to mitigate cytokine storm risks.
Microbial Engineering and Living Therapies
- Engineered Probiotics:
Modified bacteria (e.g., E. coli Nissle 1917) secrete anti-tumor molecules (e.g., IL-10) or degrade metabolic toxins (e.g., phenylalanine lyase for phenylketonuria). Synlogic’s SYNB1618 is in Phase III trials for genetic metabolic disorders. - Biosensors:
Bacteria engineered to detect disease biomarkers (e.g., tumor hypoxia) trigger localized drug release for spatiotemporal precision.
2. SynBio-Powered Clinical Applications
Cancer Immunotherapy
- Smart CAR-T Cells:
- Logic-Gated Targeting: AND-gate CAR-T cells attack dual-antigen tumors, reducing off-tumor toxicity.
- Metabolic Engineering: Enhanced mitochondrial metabolism (e.g., OXPHOS) improves CAR-T infiltration into solid tumors.
- Oncolytic Viruses:
Engineered viruses lyse tumor cells and release immune stimulants (e.g., GM-CSF) to amplify anti-cancer responses.
Genetic and Rare Diseases
- Gene Replacement/Repair:
- In Vivo Editing: AAV-delivered CRISPR systems correct RPE65 mutations in retinal dystrophy.
- mRNA Therapy: LNPs encapsulate repair mRNA to transiently fix CFTR defects in cystic fibrosis.
- Metabolic Disease Management:
Engineered hepatocytes overexpress phenylalanine hydroxylase to treat phenylketonuria.
Regenerative Medicine
- iPSC Reprogramming:
Synthetic gene circuits (e.g., OSKM factors + microRNAs) enhance iPSC differentiation into functional cardiomyocytes for heart failure treatment. - 3D Bioprinting:
Vascularized tissue scaffolds designed with SynBio secrete growth factors (e.g., VEGF) to improve organ transplant viability.
3. Challenges and Innovative Solutions
Delivery System Limitations
- Non-Viral Vectors:
Novel LNP formulations (e.g., ionizable lipids) improve mRNA delivery to non-liver organs (e.g., lungs, brain). - Bacterial Delivery:
Nanomaterial-coated engineered bacteria (e.g., gold nanoshells) enhance tumor colonization and immune evasion.
Immunogenicity and Safety
- Humanization Strategies:
CAR-T cells wrapped in human-like collagen reduce host immune rejection. - Immune Silencing:
CRISPR-Cas proteins modified at immunodominant epitopes minimize antibody neutralization.
Scalability and Cost
- Automated Biomanufacturing:
AI-driven cell culture systems (e.g., Berkeley Lights) shorten CAR-T production cycles. - Modular Genetic Parts:
Standardized BioBricks libraries reduce therapeutic vector development costs.
4. Future Trends and Strategic Opportunities
AI Integration
- AI-Assisted Design:
Deep learning models (e.g., AlphaFold for RNA) predict RNA structures to optimize CRISPR gRNA design. - Virtual Trials:
Digital twin simulations model patient responses to CAR-T therapies, streamlining preclinical testing.
Multi-Omics Precision
- Single-Cell Splicing Atlas:
Tumor microenvironment splicing variants (e.g., EGFRvIII) guide personalized CGT strategies. - Metabolomic-Epigenomic Synergy:
Dynamic epigenetic regulation (e.g., H3K27ac) enhances iPSC differentiation efficiency.
Global Collaboration and Ethics
- Open-Science Platforms:
International consortia (e.g., GP-Write) share SynBio toolkits to accelerate rare disease therapies. - Ethical Frameworks:
SynBio-Safety Level classifications balance innovation and risk.
5. Conclusion
Synthetic Biology is redefining CGT paradigms—shifting from passive repair to active programming and from single targets to system-level regulation. Despite delivery, safety, and scalability challenges, interdisciplinary collaboration (AI, nanotechnology, materials science) and global partnerships will drive three transformative breakthroughs in the next decade:
- Improved Solid Tumor Cure Rates: Logic-gated CAR-T therapies for pancreatic cancer and glioblastoma.
- Genetic Disease Cures: Single-administration in vivo editing for monogenic disorders.
- Industrialized Regenerative Medicine: Engineered organ transplants at reduced costs.
Explosive growth in this field hinges on innovation, investment, and ethical consensus, ultimately enabling a paradigm shift from disease treatment to health by design.
Data sourced from public references. For collaboration or domain inquiries, contact: chuanchuan810@gmail.com