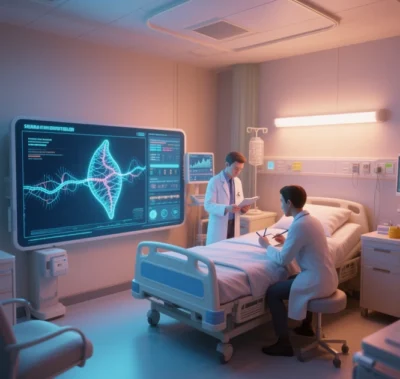
CRISPR Targeting and Precision Medicine: Revolutionizing Gene Editing to Personalized Therapies
CRISPR (Clustered Regularly Interspaced Short Palindromic Repeats) technology, a groundbreaking tool in gene editing, excels at precisely targeting specific DNA sequences. This capability aligns seamlessly with precision medicine—tailoring treatments based on individual genomic, phenotypic, and environmental profiles—to transform cancer, genetic disorders, and complex disease therapies. Below, we explore its mechanisms, applications, clinical progress, and future directions.
1. Technical Mechanisms: How CRISPR Targeting Powers Precision Medicine
Precision of Molecular Scissors
The CRISPR-Cas system uses single-guide RNA (sgRNA) to direct Cas nucleases (e.g., Cas9, Cas12a) to specific DNA sites for base insertion, deletion, or replacement. This enables precise editing of cancer-driving mutations (e.g., TP53, EGFR), repair of genetic defects (e.g., BRCA1), or regulation of immune checkpoint genes (e.g., PD-1).
Multiplex Editing and Dynamic Control
- Multiplex Targeting: Designing multiple sgRNAs allows simultaneous editing of several loci. For example, CAR-T cells can be engineered to knockout endogenous TCR genes (TRAC/TRBC) and insert neoantigen-specific TCRs to prevent graft-versus-host disease (GVHD).
- Light/Chemical Induction: Deiters Lab integrated photo-sensitive amino acids (e.g., PCK) into Cas9 for spatiotemporal editing control, minimizing off-target effects.
High-Throughput Screening
CRISPR libraries (e.g., genome-wide knockout) systematically identify genes driving tumorigenesis, drug resistance, or immune evasion. For instance, synthetic lethal gene pairs (e.g., PARP inhibitors with BRCA mutations) inform combination therapies.
2. Applications: CRISPR in Precision Medicine
Cancer Therapy: From Mutation Repair to Immune Reprogramming
- Personalized T-Cell Therapies:
- Non-Viral TCR-T Cells: PACT Pharma used CRISPR to knockout TRAC/TRBC genes in patient T cells and insert neoantigen-specific TCRs (e.g., targeting KRAS G12D), achieving tumor shrinkage in Phase I trials.
- Logic-Gated CAR-T: AND-gate circuits (e.g., CD19+CD22 dual antigen activation) restrict CAR-T activation to tumor microenvironments, reducing toxicity and achieving 92% complete remission in B-cell lymphoma.
- Tumor-Specific Gene Silencing: The i-CRISPR strategy combines CRISPR with DNA repair inhibitors to selectively kill cancer cells, validated in colon and breast cancer models.
Genetic and Rare Diseases: Correcting Mutations
- Sickle Cell Disease and β-Thalassemia: CRISPR editing of HBB in hematopoietic stem cells restores hemoglobin function (long-term efficacy shown in trials).
- Inherited Retinal Disorders: AAV-delivered CRISPR repairs CEP290 or RPE65 mutations, improving vision in patients.
Disease Modeling and Drug Development
- Organoids and PDX Models: CRISPR introduces mutations (e.g., TP53, APC in gastric cancer organoids) to mimic tumor heterogeneity and test drug sensitivity.
- Resistance Mechanisms: Knockout of EGFR T790M in lung cancer organoids reverses osimertinib resistance.
3. Clinical Progress and Challenges
Landmark Trials
- First CRISPR-T Cell Therapy: UCLA’s trial in 16 solid tumor patients demonstrated safety, with tumor shrinkage in two cases.
- Base Editing: Verve Therapeutics’ VERVE-101 uses lipid nanoparticles to deliver adenine base editors (ABE) and silence PCSK9 in the liver, lowering cholesterol in Phase I.
Technical Hurdles
- Delivery Efficiency:
- Viral Vectors: AAV8 improves liver-specific targeting.
- Non-Viral Systems: Lipid nanoparticles (LNP) or gold nanoparticles enhance in vivo editing with reduced immunogenicity.
- Off-Target Control:
- High-Fidelity Cas Variants: HypaCas9 or Cas12a reduce off-target rates by 10-100x.
- AI-Guided Design: AlphaFold for RNA optimizes sgRNA design, cutting off-target rates below 0.1%.
Ethical and Regulatory Issues
- Biosafety: Engineered microbes (e.g., carbon-capturing cyanobacteria) require multi-layered biocontainment (e.g., temperature-sensitive kill switches).
- Equity: Most CRISPR therapies rely on European genomic data; expanding to diverse populations (e.g., APOL1 nephropathy in African ancestry) is critical.
4. Future Directions
Technology Convergence
- AI-Driven Design: Deep learning predicts sgRNA-Cas9 structures to accelerate personalized therapies (e.g., tumor mutation-specific sgRNA libraries).
- Quantum-Bio Hybrids: Quantum computing simulates codon-anticodon interactions to engineer unnatural base pairs (e.g., dNaM-dTPT3).
Clinical Expansion
- In Vivo Editing: Inhalable LNPs deliver CRISPR to lungs or brain for cystic fibrosis or Huntington’s disease treatment.
- Epigenome Editing: CRISPR-dCas9 fused with epigenetic modifiers (e.g., DNMT3A) silences oncogenes without altering DNA.
Global Governance
- Data Sharing: Cross-institutional off-target databases (e.g., CRISPRme) standardize safety assessments.
- Regulatory Frameworks: The EU’s SynBio-Safety Level (SBSL) risk classification needs global coordination for gene-drive technologies.
Conclusion
CRISPR and precision medicine are evolving from “gene repair tools” to “life programming platforms.” By translating disease mechanisms into editable targets, CRISPR enables precise interventions tailored to individual pathologies. Despite delivery challenges and ethical debates, advances in AI-guided editors, base/prime editing, and delivery systems position CRISPR as the “universal language” of precision medicine. The ultimate goal transcends curing diseases—it is about proactively engineering health through designed life.
Data sourced from public references. For collaboration or domain inquiries, contact: chuanchuan810@gmail.com