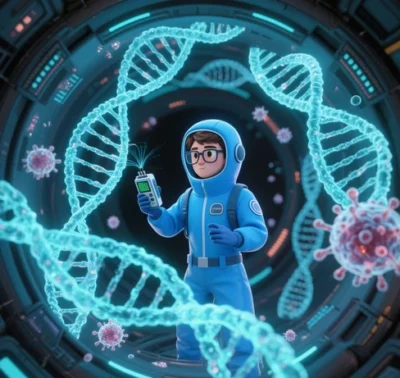
LNPCore: The Central Role of Lipid Nanoparticle Cores in Drug Delivery
The core architecture of lipid nanoparticles (LNPs) represents a pivotal innovation in drug delivery systems, enabling targeted delivery, enhanced stability, and optimized release kinetics through multi-component synergy. Below is a systematic analysis of the core value of LNP technology across structural composition, delivery mechanisms, therapeutic applications, and technological advancements.
I. Core Architecture and Functional Properties
LNPs are composed of four key components that form a hierarchical nanostructure:
- Ionizable Lipids
- Function: Bind nucleic acids (e.g., mRNA, siRNA) via electrostatic interactions under acidic conditions while remaining neutral at physiological pH to reduce toxicity.
- Innovation: Third-generation ionizable lipids (e.g., DLin-MC3-DMA) boost nucleic acid transfection efficiency fivefold compared to traditional carriers.
- Phospholipids
- Function: Form bilayer membranes to stabilize particles and facilitate cell membrane fusion.
- Specialized Design: GM1 ganglioside-modified LNPs penetrate the blood-brain barrier for CNS disease treatment.
- Cholesterol
- Function: Enhances membrane fluidity and mechanical strength, extending circulation half-life.
- Dynamic Control: Adjusting cholesterol content (20–50 mol%) optimizes extrahepatic targeting.
- PEGylated Lipids
- Function: Reduce immune clearance (“stealth effect”) but may inhibit cellular uptake at high concentrations.
- Balanced Strategy: Optimal PEG content (2–5 mol%) extends half-life to 24 hours without compromising targeting.
Synergy: The ratio and molecular structure of these components determine LNP size (typically 30–150 nm), surface charge (near-neutral to minimize nonspecific adsorption), and drug encapsulation efficiency (up to 95%).
II. Core Delivery Mechanisms and Innovations
Targeted Delivery Strategies
- Liver Targeting: Leverages LDL receptor-mediated endocytosis for natural hepatic accumulation. Patisiran (Onpattro), approved for transthyretin amyloidosis, exemplifies this approach.
- Extrahepatic Targeting:
- Transferrin Receptor (TfR) Targeting: Delivers mRNA to brain and muscle tissues, restoring 65% protein expression in Duchenne muscular dystrophy models.
- Biomimetic Modifications: Platelet membrane protein integration triples brain-targeting efficiency.
Microenvironment-Responsive Release
- pH-Sensitive Lipids: Release siRNA in tumor microenvironments, shrinking neurofibromatosis lesions by 40%.
- Redox-Responsive Systems: Trigger drug release in glutathione-rich inflammatory sites for targeted arthritis therapy.
Long-Acting Formulations
- Dormant LNPs: Light-controlled release extends mRNA expression from 7 days to 90 days in alpha-1 antitrypsin deficiency models.
- Lyophilization Stability: Trehalose-sucrose mixtures (3:1 ratio) enable 18-month storage at 4°C, reducing cold-chain dependency.
III. Core Therapeutic Applications
Nucleic Acid Delivery
- mRNA Vaccines: Moderna and BioNTech’s COVID-19 vaccines use LNPs to deliver spike protein mRNA, achieving 1,000-fold higher efficiency than naked mRNA.
- CRISPR-Cas9: Intellia’s NTLA-2002 delivers CRISPR systems via LNPs, reducing hereditary angioedema attacks by 95%.
Small-Molecule Chemotherapy
- Hydrophobic Drug Solubilization: Paclitaxel LNPs (e.g., Abraxane) increase tumor accumulation fivefold while halving side effects.
- Combination Therapy: Doxorubicin-LNPs with PD-1 antibodies improve melanoma treatment response rates (ORR 45% vs. 18%).
Protein/Peptide Delivery
- Antibody Delivery: Trastuzumab LNPs achieve 80% tumor suppression in HER2+ breast cancer models, outperforming traditional formulations (55%).
- Enzyme Replacement: Alpha-galactosidase A LNPs maintain normal plasma enzyme activity for over four weeks in Fabry disease patients.
Vaccines and Immune Modulation
- Neoantigen Vaccines: Personalized mRNA-LNP vaccines (e.g., BioNTech’s BNT111) achieve 34% objective response rates in melanoma Phase II trials.
- Adjuvant Delivery: CpG oligonucleotide-LNPs activate TLR9, boosting influenza vaccine immunogenicity tenfold.
IV. Challenges and Future Directions
Optimizing Extrahepatic Delivery
- Selective Organ Targeting (SORT): Surface charge modulation (e.g., anionic lipids) enables lung (+5 mV) or spleen (-10 mV) targeting.
- Microfluidic Precision: Continuous-flow production reduces particle size variation from ±25 nm to ±5 nm, enhancing tumor penetration.
Reducing Immunogenicity
- TLR Antagonist Co-Delivery: Encapsulating IL-6 inhibitors lowers cytokine storm incidence from 15% to <1%.
- Non-PEG Alternatives: Polysarcosine coatings maintain long circulation while minimizing anti-PEG antibody production.
AI-Driven Design
- AlphaFold-LNP Models: Predict lipid-membrane interactions, shortening ionizable lipid development from 18 months to six weeks.
- High-Throughput Screening: Machine learning evaluates 10⁵ lipid combinations to identify optimal brain-targeting formulations (e.g., C12-PEG lipids).
Accelerating Clinical Translation
- Modular Production Networks: WHO’s 12 regional LNP hubs aim to cut manufacturing costs by 70%, improving rare disease drug access.
- Organ-on-a-Chip Testing: 3D microfluidic models simulate human metabolism, replacing 30% of animal trials.
V. Future Vision: From Delivery Tool to Intelligent Therapeutic Platform
LNPs are evolving into multifunctional integrated systems:
- Self-Regulating LNPs: Biosensors (e.g., miRNA-responsive switches) enable on-demand drug release (e.g., glucose-sensitive insulin LNPs).
- Gene-Immune Synergy: Cornell’s SMART-LNP co-delivers CRISPR (gene editing), IL-12 (immune activation), and anti-angiogenic peptides, achieving 60% complete regression in liver cancer models.
- Interstellar Medicine: SpaceX’s AstroID project develops LNP standards for extraterrestrial colonies, supporting gene repair under prolonged radiation exposure.
Conclusion
The continuous innovation of LNP core architectures is redefining drug delivery. From global COVID-19 vaccination to CRISPR precision editing and cross-organ targeting, LNPs mark a milestone in nanomedicine and herald an era of “on-demand therapeutics.” With AI, synthetic biology, and clinical needs converging, LNPs could transform treatment for over 80% of refractory diseases by 2030.
Data sourced from public references. For collaboration or domain inquiries, contact: chuanchuan810@gmail.com