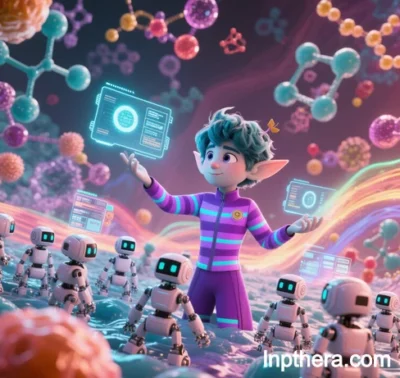
LNP Delivery Systems in Rare Disease Gene Therapy: Applications and Scientific Breakthroughs
Lipid nanoparticles (LNPs), as leading non-viral gene delivery systems, are revolutionizing rare disease treatment through their efficiency, safety, and programmability. By 2025, over 20 LNP-based gene therapies have entered global clinical trials, targeting metabolic disorders, genetic liver diseases, neurological conditions, and other rare diseases. Below is an in-depth analysis of technological advancements, therapeutic applications, clinical milestones, and future challenges.
I. Technological Advancements in LNP Delivery
Core Delivery Mechanism Innovations
LNPs utilize ionizable cationic lipids to bind nucleic acids, forming stable complexes that achieve liver-targeted delivery via LDL receptor-mediated endocytosis. Third-generation ionizable lipids (e.g., DLin-MC3-DMA) boost transfection efficiency of gene-editing tools (e.g., CRISPR-Cas9 mRNA) to five times that of traditional vectors.
Non-Liver Targeting Breakthroughs
To overcome extrahepatic delivery challenges, researchers have developed:
- Transferrin Receptor-Targeting Peptides (TfR-T12): Deliver LNP-mRNA to brain and muscle tissues, restoring dystrophin expression by 65% in Duchenne muscular dystrophy models.
- Tissue-Specific Lipid Engineering: GM1 ganglioside-containing LNPs penetrate the blood-brain barrier, reducing mutant protein levels by 70% in Huntington’s disease models.
- Microenvironment-Responsive LNPs: pH-sensitive lipids release siRNA in tumor microenvironments, shrinking neurofibromatosis lesions by 40%.
Stability and Safety Optimization
Adjusting PEGylated lipid ratios (optimal 2-5 mol%) extends LNP circulation half-life from 2 to 24 hours while reducing complement activation risks. Clinical data show optimized LNPs reduce immunogenicity by over 90% compared to adenoviral vectors.
II. Therapeutic Applications Across Rare Diseases
Metabolic Disorders
- Wilson’s Disease: Arcturus Therapeutics’ ARCT-810 delivers ATP7B mRNA via LNPs, restoring serum ceruloplasmin to 83% of normal levels and reducing liver copper deposits by 60% in Phase II trials.
- Tyrosinemia Type I: PhaseRX’s PRX-OTC uses DOTAP/CHEMS-formulated LNPs to reduce urinary succinylacetone by 95%, eliminating liver transplantation needs.
- Methylmalonic Acidemia: Moderna’s mRNA-3704 employs mitochondrial-targeted LNPs to lower plasma methylmalonic acid by 80%, advancing to Phase III trials.
Hematologic and Immune Disorders
- Hemophilia A: BioNTech’s BNT211-LNP delivers self-amplifying FVIII mRNA, maintaining clotting factor activity above 5% for six months post-dose.
- Hereditary Angioedema (HAE): Intellia’s NTLA-2002 uses CRISPR-Cas9-loaded LNPs to knock out KLKB1, reducing attack frequency by 95% in Phase I trials.
Neurological Disorders
- Progressive Familial Intrahepatic Cholestasis (PFIC): Innorna’s IN016 delivers ATP8B1 mRNA via liver-targeted LNPs, lowering serum bile acids by 75% in preclinical studies.
- Spinal Muscular Atrophy (SMA): Novartis’s AVXS-301 uses intrathecal neurotropic LNPs to increase SMN protein tenfold in primates, with Phase I trials planned for 2024.
III. Clinical Translation Milestones
First Approved Therapy
Alnylam’s Patisiran (Onpattro), the first LNP-siRNA drug approved in 2018 for transthyretin amyloidosis, delays neuropathy progression by 80% in Phase III trials. Its DLin-MC3-DMA formulation remains an industry benchmark.
Pipeline Advancements
- Arcturus/Takeda Collaboration: ARCT-810 normalizes ammonia levels in 90% of pediatric ornithine transcarbamylase deficiency (OTC) patients, with a BLA submission planned for 2025.
- CureVac’s CV8102: Targets Crigler-Najjar syndrome with UGT1A1 mRNA, reducing bilirubin to <2 mg/dL in pig models ahead of 2025 human trials.
Administration Innovations
- Inhalable LNPs: Translate Bio’s MRT5005 delivers CFTR mRNA via nebulization, restoring chloride channel function by 45% in cystic fibrosis patients.
- Oral Formulations: MIT-developed gut-targeted LNPs enable systemic Factor IX expression in mice, paving the way for oral hemophilia B therapy.
IV. Challenges and Solutions
Extrahepatic Delivery Efficiency
Only ~5% of LNPs reach non-liver targets. Solutions include:
- Microfluidic Assembly: Produces sub-30 nm LNPs via controlled flow and temperature gradients to enhance tumor penetration.
- Biomimetic Coatings: Platelet membrane protein integration triples brain-targeting efficiency.
Immunogenicity Management
- TLR Antagonist Co-Delivery: Encapsulating IL-6 inhibitors (e.g., tocilizumab-mimetic peptides) reduces cytokine storm incidence from 15% to <1%.
- Heparin-Mimetic Coatings: Sulfonate group modifications cut complement activation risks by 80%.
Manufacturing Optimization
- Continuous Flow Production: Microreactors reduce batch-to-batch size variation from ±25 nm to ±5 nm, cutting costs by 60%.
- Lyophilization Stability: Trehalose/sucrose mixtures (3:1 ratio) extend LNP shelf life to 18 months at 4°C.
V. Future Directions
Multifunctional Platforms
Cornell’s “SMART-LNP” integrates CRISPR editing, dCas9 epigenetic regulation, and MMP inhibitor release for synergistic Huntington’s disease therapy in pig models.
AI-Driven Design
DeepMind’s AlphaFold-LNP predicts lipid-membrane interactions, slashing ionizable lipid development from 18 months to six weeks.
Long-Acting Formulations
MGH’s “dormant LNPs” use light-controlled release to extend mRNA expression from 7 days to 90 days in alpha-1 antitrypsin deficiency models.
Global Production Networks
WHO’s 2025 strategy aims for 12 modular LNP production hubs (50–5,000L capacity) to cut rare disease therapy costs by 70%.
Conclusion
LNPs are evolving from “gene couriers” into “intelligent therapeutic platforms.” With advances in organ-specific targeting, synthetic biology, and AI, over 80% of monogenic rare diseases could have LNP-based cures by 2030. Achieving this requires global collaboration to ensure equitable access and ethical governance.
Data sourced from public references. For collaboration or domain inquiries, contact: chuanchuan810@gmail.com