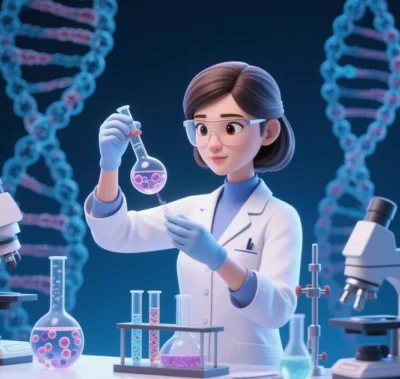
SynthmRNA vs. Natural mRNA: A Comparative Analysis
1. Definition and Basic Structure
- Natural mRNA: Transcribed from nuclear DNA, it carries genetic information for protein synthesis. Its structure includes a 5′ cap, 5′ untranslated region (UTR), open reading frame (ORF), 3′ UTR, and poly(A) tail. Natural nucleotide modifications (e.g., methylation, pseudouridine) are site-specific and occur at low rates, primarily regulating translation efficiency and stability.
- Synthetic mRNA (SynthmRNA): Artificially synthesized via in vitro transcription (IVT), it mimics natural mRNA structure but incorporates engineered modifications (e.g., m1Ψ, 5mC, 2′-fluoro nucleotides) or unnatural bases (e.g., expanded genetic alphabets) to enhance stability, translation efficiency, and immunogenicity control.
2. Key Differences
Dimension | Natural mRNA | Synthetic mRNA |
---|---|---|
Source | Naturally transcribed from nuclear DNA. | Artificially synthesized using IVT (e.g., T7 RNA polymerase). |
Nucleotide Modifications | Natural modifications (e.g., m6A, pseudouridine) at low, site-specific rates. | Engineered modifications (e.g., m1Ψ, 5mC) or unnatural bases at high rates. |
Stability | Short half-life due to nuclease sensitivity. | Enhanced stability via poly(A) tail elongation and UTR optimization. |
Immunogenicity | Low (self-molecule), but triggers innate immunity if mislocalized. | High (unmodified), but reduced via modifications (e.g., m1Ψ inhibits TLR recognition). |
Translation Efficiency | Moderate, governed by native UTRs and codon bias. | Optimized UTRs (e.g., α-globin UTR), codon usage, and 5′ cap boost efficiency. |
Delivery | Naturally trafficked within cells (nucleus to cytoplasm). | Requires delivery systems (e.g., LNPs, viral-like particles) for cellular uptake. |
3. Engineering Innovations in Synthetic mRNA
3.1 Chemical Modifications
- Nucleotide Substitutions:
- Pseudouridine (Ψ) and Methylation: m1Ψ replaces uridine to reduce immune detection and enhance translation (key to Moderna/BioNTech vaccines).
- Thiolation (s2U): Improves stability against RNase degradation.
- Unnatural Bases: Enable site-specific tagging via click chemistry for intracellular tracking.
- Structural Optimization:
- 5′ Cap and Poly(A) Tail: Anti-reverse cap analogs (ARCA) and extended poly(A) tails enhance ribosome recruitment.
- UTR Design: Machine learning predicts high-efficiency UTRs (e.g., CMV or α-globin UTR) to boost translation.
3.2 Delivery Systems
- Lipid Nanoparticles (LNPs): Charge-driven encapsulation enables systemic delivery (e.g., COVID-19 vaccines).
- Viral-Like Particles (VLPs): Self-assembling capsid proteins offer biocompatibility and targeting.
- Exosome Delivery: Engineered exosomes with surface ligands target specific tissues (e.g., CNS).
4. Functional and Application Comparisons
Application | Natural mRNA Role | Synthetic mRNA Applications |
---|---|---|
Protein Synthesis | Essential for physiological functions (enzymes, structural proteins). | Replaces defective gene products (e.g., CFTR protein in cystic fibrosis). |
Disease Therapy | Not directly therapeutic. | Vaccine development (e.g., COVID-19), rare disease treatment (e.g., SMA). |
Cell Reprogramming | Maintains cellular homeostasis. | Generates iPSCs or transdifferentiates cells (e.g., CAR-T engineering). |
Dynamic Control | Regulated by native signaling pathways. | Light-/temperature-responsive switches (e.g., CRISPR-SLIDE) enable precise spatiotemporal expression. |
5. Challenges and Future Directions
5.1 Limitations of Natural mRNA
- Instability: Rapid degradation by RNases limits in vitro applications.
- Immune Evasion: Exogenous delivery triggers innate immune responses (e.g., IFN-α secretion).
5.2 Bottlenecks in Synthetic mRNA
- Delivery Efficiency: LNPs exhibit liver tropism and poor blood-brain barrier penetration.
- Long-Term Safety: High doses or repeated use may induce autoimmune reactions.
- Production Costs: Complex purification (e.g., HPLC) increases therapy costs.
5.3 Future Technological Convergence
- AI-Driven Design: AlphaFold predicts mRNA-ribosome interactions to optimize translation.
- Epigenetic Integration: Combines dCas9-DNMT3A with synthetic mRNA for synergistic regulation.
- Quantum Computing: Simulates mRNA folding dynamics to design ultra-stable constructs.
6. Conclusion
Synthetic mRNA surpasses natural mRNA in stability, efficiency, and controllability, revolutionizing vaccine development, gene therapy, and synthetic biology. Challenges in immunogenicity, delivery precision, and cost persist. Future integration with AI, quantum computing, and novel delivery systems (e.g., VLPs) may bridge the gap from “protein replacement” to “cellular reprogramming.”
Data sourced from public references. For collaboration or domain inquiries, contact: chuanchuan810@gmail.com