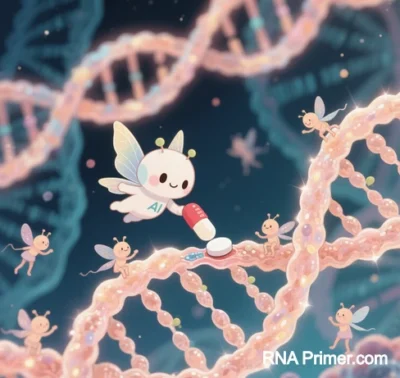
RNA Primers: Core Roles and Applications in DNA Replication, PCR, and Reverse Transcription
RNA primers are indispensable tools in molecular biology, playing critical roles in DNA replication, PCR amplification, and reverse transcription. Their unique chemical properties and biological functions underpin genome stability, genetic engineering, and precision medicine. Below, we explore their practical applications and transformative potential across key domains.
I. DNA Replication: The “Molecular Key” to Genetic Inheritance
- Molecular Basis of Replication Initiation
DNA polymerases cannot synthesize DNA de novo and require RNA primers to provide a 3′-OH group for elongation. Primase synthesizes 10-20 nt RNA primers in prokaryotes and eukaryotes, enabling DNA strand extension.
Biological Significance: This mechanism ensures high replication fidelity by preventing random errors from free 3′ ends. - Dynamic Regulation of Replication Forks
- Leading Strand: Continuous synthesis requiring only one RNA primer.
- Lagging Strand: Discontinuous synthesis via Okazaki fragments (each requiring an RNA primer), later ligated into a complete strand.
Innovation: In eukaryotes, RNase H2 and FEN-1 nucleases excise RNA primers, while DNA ligase seals gaps for seamless repair.
- Solving the Telomere Replication Problem
Telomerase uses its intrinsic RNA template to synthesize telomeric repeats, with the RNA component acting as a primer to address the “end replication problem.”
Clinical Relevance: Dysregulated telomerase activity is linked to cancer and progeria, making it a therapeutic target.
II. PCR Technology: From Basic Amplification to Advanced Innovations
- Dominance of DNA Primers in Traditional PCR
- Design principles: 18-24 nt length, 40-60% GC content, avoidance of secondary structures and dimers.
- Applications: Gene cloning, mutation detection, pathogen diagnosis.
- Specialized Roles of RNA Primers
- RNA-Primed PCR: Uses rTth DNA polymerase’s reverse transcriptase activity to amplify unknown DNA regions directly with RNA primers.
- Enables genome walking without pre-designed primers.
- Requires Mn²⁺ optimization for efficiency, albeit lower than standard PCR.
- RT-qPCR: Oligo(dT) and random primers enhance sensitivity for low-abundance RNA detection during reverse transcription.
- Third-Generation PCR Innovations
Digital PCR and microfluidic chips integrated with RNA primer labeling achieve single-molecule nucleic acid detection, offering ultra-high sensitivity for liquid biopsies and rare mutation screening.
III. Reverse Transcription: From cDNA Synthesis to Precision Medicine
- Mechanism of Reverse Transcriptase
- Synthesizes cDNA from RNA templates using dNTPs and primers (typically DNA) providing a 3′-OH group.
- Key enzymes: AMV reverse transcriptase (high fidelity), MMLV reverse transcriptase (thermostable).
- Primer Selection Strategies
Primer Type Advantages Limitations Oligo(dT) Eukaryotic mRNA (Poly-A tail) Requires intact RNA Random Hexamers Degraded/prokaryotic RNA Generates short cDNA fragments Gene-Specific Targets known sequences Requires prior sequence info Mixed Primers Balances coverage (e.g., scRNA-seq) Requires ratio optimization - Clinical Breakthroughs
- HIV Viral Load Monitoring: Reverse transcription-digital PCR detects down to 1 copy/μL.
- Liquid Biopsy: ctRNA reverse transcription identifies EGFR T790M mutations to guide targeted cancer therapy.
IV. RNA vs. DNA Primers: Key Differences and Selection Logic
Property | RNA Primers | DNA Primers |
---|---|---|
Synthesis | Enzymatically synthesized in vivo | Chemically synthesized in vitro |
Stability | RNase-sensitive (requires inhibitors) | Nuclease-resistant |
Applications | Native DNA replication, telomere extension | PCR, sequencing, gene editing |
Cost/Throughput | Enzyme-dependent (higher cost) | Scalable synthesis (low cost) |
Customizability | Limited by natural enzymes | Modifiable (e.g., fluorescent tags, phosphorothioates) |
V. Future Directions and Innovations
- Synthetic Biology Tools
- Self-Excising Primers: Enable in vivo removal and seamless ligation to boost gene-editing efficiency.
- Light-Controlled Systems: Spatiotemporal replication regulation via photosensitive primer modifications.
- Nanodelivery Integration
Lipid nanoparticles (LNPs) co-deliver CRISPR-Cas9 and RNA primers to target tissues for precision gene therapy. - AI-Driven Design
Deep learning models (e.g., DeepPrimer) predict RNA primer binding affinity, optimize cross-species compatibility, and reduce development cycles by 90%. - Clinical Translation Challenges
- Enhancing RNA primer stability in bodily fluids (e.g., 2′-fluoro modifications).
- Engineering universal reverse transcriptase mutants to minimize primer dimer formation.
Conclusion
RNA primers are not merely “molecular switches” for genetic information transfer but also versatile tools in genetic engineering and precision medicine. With advancements in synthetic biology and nanotechnology, their applications are expanding from basic research to clinical therapies and environmental monitoring. Chinese research teams are leading innovations in CRISPR-Cas9 delivery systems and high-throughput primer design, signaling an impending technological revolution in this field.
Data sourced from public references. For collaboration or domain inquiries, contact: chuanchuan810@gmail.com