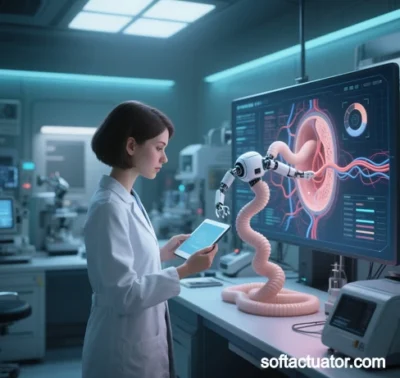
Soft Actuators: Recent Advances in Medical, Rehabilitation, and Bionic Robotics
Soft actuators, with their flexibility, biocompatibility, and biomimetic motion capabilities, are driving transformative advancements in medical, rehabilitation, and bionic robotics. Below is an exploration of their breakthroughs across these domains.
I. Medical Applications
1. Minimally Invasive Surgery & Endoscopic Tools
- Adaptive Surgical Instruments: Pneumatically driven soft actuators (e.g., silicone bellows structures) enable flexible forceps and catheters to navigate narrow anatomical pathways (e.g., blood vessels, intestines) for precision operations. Deformable soft grippers avoid mechanical damage to delicate tissues, enabling “scarless” procedures in neurosurgery and cardiovascular interventions.
- Smart Endoscopes: Fiber-optic-integrated soft actuators detect tissue stiffness in real time and adjust grasping force. Harvard’s magnetically controlled capsule endoscope uses soft micro-arms for biopsies or targeted drug delivery.
2. Targeted Drug Delivery Systems
- Environment-Responsive Carriers: Light- or pH-sensitive soft actuators (e.g., liquid crystal elastomers) trigger drug release in tumor microenvironments. Programmable soft nanobots deform to breach vascular barriers, delivering chemotherapy directly to cancer cells.
- Biodegradable Actuators: PLA-based soft actuators (developed by Peking University) autonomously degrade after fallopian tube drug delivery, eliminating follow-up surgeries.
3. Biomimetic Artificial Organs
- Cardiac Assist Devices: Hydraulic soft pumps mimic myocardial contractions (e.g., Harvard’s “soft heart”) to support blood circulation in heart failure patients, outperforming traditional metal pumps in biocompatibility.
- Artificial Lungs & Kidneys: Porous elastomer-based actuators simulate gas exchange in alveoli or renal tubules, offering temporary organ replacement solutions.
II. Rehabilitation Innovations
1. Wearable Exoskeletons & Assistive Devices
- Neurological Rehabilitation Exoskeletons: Pneumatic artificial muscle (PAM)-based soft exoskeletons (e.g., ReWalk’s knee brace) provide gait training support for stroke or spinal injury patients. Lightweight designs (< kg) surpass rigid exoskeletons.
- Smart Rehabilitation Gloves: Shape-memory alloy (SMA) actuators mimic finger tendon movements. MIT’s “ExoGlove” uses thermal actuation for personalized grip rehabilitation.
2. Brain-Machine Interfaces & Neuromodulation
- Flexible Neural Electrodes: Hydrogel-based soft electrodes conform to the brain’s surface, enabling high-density neural signal capture and stimulation. Caltech’s “Neurogrid” decodes macaque motor intent via soft microelectrode arrays.
- Closed-Loop Rehabilitation: ETH Zurich’s bionic prosthetics combine EMG signals with electroactive polymer (EAP) actuators for tactile-motor feedback control.
3. Elderly Care & Functional Augmentation
- Assistive Clothing: Dielectric elastomer actuators (DEA) embedded in clothing provide joint assistance for the elderly. Xenoma’s smart carewear reduces caregiver back strain during patient transfers.
III. Bionic Robotics & Design
1. Biomimetic Motion
- Underwater Robots: Octopus-inspired soft grippers (e.g., Festo’s OctopusGripper) use multi-chamber pneumatic actuation for complex grasping and propulsion in deep-sea exploration.
- Flying Insect Robots: Harvard’s “RoboBee” employs PVDF actuators to mimic bee wing flapping, achieving autonomous takeoff and hovering.
2. Environmental Adaptation
- Terrain Robots: Origami-structured soft actuators (e.g., MIT’s Origami Crawler) fold to traverse sand, snow, or disaster zones.
- Self-Healing Robots: Soft actuators with self-healing elastomers recover mechanical properties post-damage via heat or light, extending durability in extreme environments.
3. Swarm Robotics & Smart Materials
- Modular Soft Robots: KAIST’s “Soft Robotics Block” (SoBL) self-assembles via magnetic coupling and pneumatic actuation for collaborative tasks.
- 4D-Printed Structures: Shape-memory polymer actuators morph over time or temperature (e.g., self-deploying stents or solar panel cleaners).
IV. Challenges & Future Directions
1. Material & Energy Limitations
- Biocompatibility: Current materials (e.g., silicone) risk fibrosis during long-term implantation. Next-gen bioactive hydrogels aim to induce tissue regeneration.
- Energy Efficiency: Transition from external pumps to self-powered systems using biofuel cells or triboelectric nanogenerators.
2. Intelligent Systems
- Embedded Sensing: Integrate flexible strain sensors (e.g., graphene/PDMS composites) for real-time deformation feedback.
- AI-Driven Control: Reinforcement learning optimizes nonlinear dynamics (e.g., Boston Dynamics’ “SoftGym” platform).
3. Scalability & Ethics
- Low-Cost Manufacturing: Multi-material DLP printing reduces production costs to of traditional methods.
- Ethical Standards: Establish global safety protocols for traceability and emergency response in medical soft robotics.
Conclusion
Soft actuators are redefining the boundaries of medicine, rehabilitation, and bionics through material-structure-actuation synergy. In healthcare, they enable minimally invasive and adaptive interventions. In rehabilitation, they restore mobility via neural integration. In robotics, they unlock lifelike environmental adaptability. Over the next decade, interdisciplinary advances (e.g., synthetic biology, quantum computing) aim to achieve:
- By 2030: First lifelong implant of a fully soft artificial heart.
- By 2035: Self-learning bionic swarm robotics systems.
This technological wave will reshape human-machine collaboration and fuse life sciences with engineering.
Data sourced from public references. For collaboration or domain inquiries, contact: chuanchuan810@gmail.com