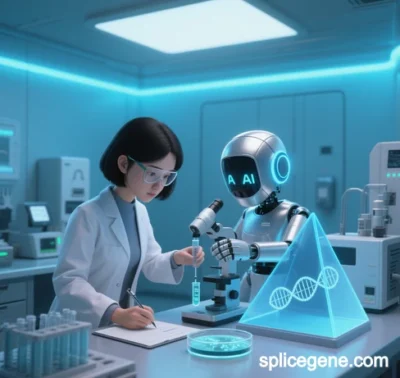
Splice Genes: The Architecture and Functional Core of Eukaryotic Genomes
Splice genes—characterized by protein-coding exons interspersed with non-coding introns—are a hallmark of eukaryotic genomes. These genes require RNA splicing to remove introns and generate mature mRNA, serving as both a regulatory hub for gene expression and an evolutionary driver of biological complexity and diversity. Below is a systematic analysis of their structural features, molecular mechanisms, biological roles, and evolutionary significance.
I. Core Structural Features
1. Exon-Intron Alternation
- Exons: Protein-coding sequences (~1–5% of total gene length).
- Introns: Non-coding sequences (majority of gene length), removed post-transcription. Some introns regulate splicing or gene expression.
2. Splice Sites
- Conserved Sequences:
- Donor Site: 5′ GT at intron start.
- Acceptor Site: 3′ AG at intron end (GT-AG rule).
- Branch Site: Located upstream of the acceptor site (e.g., YNYURAC), critical for spliceosome assembly.
3. Splice Signal Diversity
- Strong/Weak Sites: Strong sites match conserved motifs (e.g., AG-GT); weak sites bind regulatory proteins (e.g., SRp20) for alternative splicing.
- Exon Skipping/Intron Retention: Generates diverse mRNA isoforms.
4. The Spliceosome Machinery
- Composition: Five small nuclear ribonucleoproteins (snRNPs: U1, U2, U4, U5, U6) and hundreds of auxiliary proteins (megadalton-scale).
- Dynamic Assembly: Undergoes stepwise catalytic cycles (e.g., branch site recognition, transesterification) to excise introns.
II. Biological Functions & Evolutionary Significance
1. Protein Diversity Expansion
- Alternative Splicing: A single gene produces multiple mRNA isoforms (e.g., 3–4 isoforms per human gene). Notable examples:
- DSCAM (fruit fly): 38,000 isoforms regulate neuronal connectivity.
- TPM1: Exon combinations modulate actin filament assembly.
- Non-Coding RNAs: Intronic transcripts (e.g., lncRNAs, miRNAs) participate in epigenetic regulation.
2. Precision Gene Regulation
- Transcription-Splicing Coupling: RNA Pol II’s C-terminal domain (CTD) recruits splicing factors (e.g., SRp20) for spatiotemporal coordination.
- Quality Control:
- Nonsense-Mediated Decay (NMD): Degrades mRNA with premature stop codons.
- Proofreading: Spliceosome校对机制 ensures splicing fidelity.
3. Genomic Evolution & Adaptation
- Exon Shuffling: Introns enable exon recombination/transposition, accelerating functional protein evolution.
- Intron Functionalization: Some introns evolve into regulatory elements (enhancers/silencers) or non-coding RNAs, driving species-specific traits.
4. Disease Links & Medical Applications
- Splicing Errors in Disease:
- Cancer: BRCA1 splicing defects impair DNA repair (breast cancer risk).
- Neurodegeneration: Aberrant MAPT splicing links to Tau aggregation (Alzheimer’s).
- Therapeutic Targets: Antisense oligonucleotides (ASOs) correct splicing errors (e.g., SMN2 modulation for spinal muscular atrophy).
III. Research Tools & Technological Frontiers
1. Splice Site Prediction
- Machine Learning: Tools like SpliceMachine (SVM) and GeneSplicer (Markov model) predict sites with >90% accuracy using sequence features.
- Deep Learning: CNN-based models integrate multi-omics data (chromatin accessibility, RBP footprints) for enhanced precision.
2. Single-Molecule & Structural Analysis
- Live-Cell Imaging: CRISPR-tagged MS2/MCP systems track spliceosome assembly and pre-mRNA processing in real time.
- Cryo-EM: Resolves high-resolution structures of spliceosome catalytic states (e.g., U2/U6 snRNP conformational shifts).
3. Synthetic Biology Interventions
- Engineered Splicing Factors: dCas9 fusions (e.g., CasRx) target specific splicing events for gene therapy.
- Quantum Dot Probes: Gold nanoparticle-enhanced Raman spectroscopy monitors splicing dynamics.
IV. Open Questions & Challenges
1. Spatiotemporal Regulation
Deciphering tissue-specific splicing factors (e.g., NOVA, FOX) and their interplay with 3D chromatin architecture.
2. Splicing Fidelity & Aging
Mechanisms underlying age-related splicing errors and their role in age-associated diseases.
3. Prokaryotic Splicing Evolution
Functional homology of intron-like structures in archaea/bacteria remains debated.
Conclusion
Splice genes are central to eukaryotic complexity, where exon-intron architecture acts as both a “molecular switch” for gene expression and an “evolutionary playground” for genomic innovation. From alternative splicing to disease therapies, and from evolutionary mechanisms to synthetic biology, this field continues to drive life science frontiers. As the spliceosome’s dynamic remodeling metaphorizes: Life’s complexity arises from precise “molecular tailoring,” and science seeks to decode its design principles.
Data sourced from publicly available references. For collaborations or domain inquiries, contact: chuanchuan810@gmail.com.