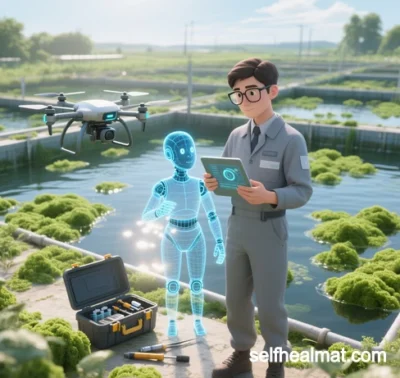
Self-Healing Materials in Life Sciences: Definitions, Mechanisms, and Applications
Self-healing materials are intelligent substances capable of autonomously repairing physical damage (e.g., cracks, fractures) or functional degradation through intrinsic or extrinsic mechanisms. In life sciences, this concept draws inspiration from biological self-repair systems—such as skin wound healing and DNA damage repair—to imbue materials with lifelike properties via chemical, physical, or biological strategies. The core objectives are to extend material lifespan, enhance safety, and expand applications in biomedicine, soft robotics, and energy storage.
Key Mechanisms and Classifications
1. Classification by Repair Mechanisms
- Extrinsic Self-Healing:
Relies on pre-embedded repair agents (e.g., microcapsules, microvascular networks) released upon mechanical damage. For example, polymer composites with microcapsules release monomers upon rupture, filling cracks via polymerization. These materials are used in orthopedic implants and dental composites but face limitations in mechanical robustness. - Intrinsic Self-Healing:
Utilizes dynamic chemical bonds (e.g., hydrogen bonds, coordination bonds, reversible covalent bonds) for autonomous repair. Supramolecular polymer hydrogels, for instance, restore structural integrity through hydrogen bond reorganization, making them ideal for tissue engineering and drug delivery.
2. Bioinspired Repair Strategies
- Cell-Mimetic Responses: Synthetic biology integrates engineered microbes (e.g., E. coli) into materials to secrete repair proteins or metabolites upon damage.
- Vascular Network Mimicry: Microchannel systems resembling blood vessels deliver healing agents to damaged areas, akin to biological circulation.
Core Applications in Life Sciences
1. Biomedical Engineering
- Tissue Engineering Scaffolds: Self-healing hydrogels mimic the extracellular matrix (ECM), supporting stem cell proliferation. Hydrogels with hydroxyapatite-binding peptides demonstrate dynamic repair in bone defect regeneration.
- Smart Drug Delivery: Damage-triggered microcapsules release drugs or growth factors for targeted therapies. Acid-responsive materials in tumor microenvironments release chemotherapeutic agents.
- E-Skin and Wearables: Flexible self-healing materials (e.g., octopus-inspired synthetic proteins) endure mechanical stress while monitoring physiological signals and enabling real-time repair.
2. Soft Robotics
- Adaptive Actuators: Bioinspired protein-based materials developed by Max Planck Institute and Penn State repair mechanical damage in 1 second, enabling pneumatic-driven soft grippers for precision tasks in food and pharma industries.
- Environmental Interfaces: Self-healing coatings protect robotic sensors from corrosion or wear, enhancing durability in harsh environments.
3. Energy and Sustainability
- Self-Healing Batteries: The EU’s BATTERY 2030+ initiative proposes sensors to detect damage and trigger electrolyte self-repair, improving energy storage safety and longevity.
- Eco-Materials: Hydrophobic self-healing coatings inspired by plant leaves prevent microbial adhesion, reducing industrial maintenance needs.
Challenges and Future Directions
1. Technical Limitations
- Strength-Efficiency Trade-off: Current materials achieve repair strengths of 2–23 MPa, below biological tissues like tendons (100 MPa).
- Environmental Adaptability: Materials must maintain stability under physiological conditions (pH, temperature shifts), yet most designs remain lab-confined.
2. Interdisciplinary Innovations
- Synthetic Biology Integration: CRISPR-engineered microbes detect damage and activate repair pathways. Fluorescent protein-expressing bacteria, for example, mark and guide repair in damaged zones.
- AI and Quantum Computing: Machine learning predicts dynamic bond reorganization pathways, accelerating molecular design.
3. Ethics and Biosafety
- Biocompatibility Risks: Microcapsules in extrinsic materials may trigger immune responses, necessitating biodegradable or inert carriers.
- Ecological Impact: Long-term environmental behavior (e.g., microbial leakage) requires multi-scale modeling for biohybrid materials.
Conclusion and Outlook
Self-healing materials are evolving from passive protection to active adaptation in life sciences, bridging the gap between non-living matter and biological intelligence. Future breakthroughs driven by synthetic biology, quantum computing, and materials science include:
- Multi-Scale Repair: Integrating molecular-level DNA repair (e.g., CRISPR-guided gene correction) with macroscopic structural regeneration.
- Life-Mimetic Systems: Developing “living materials” with metabolism, growth, and self-healing capabilities, blurring the boundaries between biology and technology.
Advancing this field demands not only innovation but also ethical frameworks to balance biosafety and technological ambition.
Data sourced from publicly available references. For collaborations or domain inquiries, contact: chuanchuan810@gmail.com.