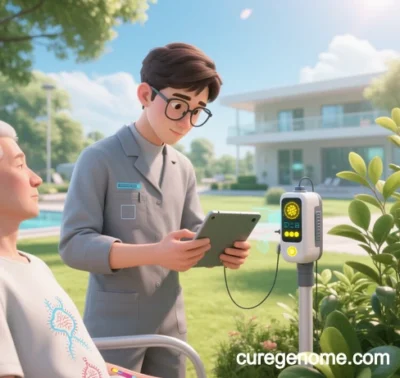
Cure Genome: Permanent Genomic Defect Repair via Gene-Editing Technologies
Breakthroughs in gene-editing technologies, particularly CRISPR-Cas9 system optimizations, have enabled precise strategies to permanently repair pathogenic genomic defects. By directly modifying DNA sequences or regulating gene expression, these approaches address genetic mutations at their source, offering treatments for hereditary diseases, cancers, and more. Below are the most advanced strategies and their applications:
I. Double-Strand Break (DSB)-Dependent Repair Strategies
1. Non-Homologous End Joining (NHEJ) & Homology-Directed Repair (HDR)
- NHEJ: Cas9-induced DSBs are repaired via the cell’s intrinsic NHEJ mechanism, enabling gene knockout or small insertions/deletions (indels). In Duchenne muscular dystrophy (DMD), CRISPR-Cas9 excises mutated exons (e.g., exon 51), restoring the dystrophin reading frame and improving muscle function.
- HDR: Uses donor DNA templates for precise repair of single-base mutations or large replacements. In hereditary tyrosinemia type I mouse models, intravenous CRISPR-Cas9 and donor templates repaired Fah gene point mutations in hepatocytes, reversing disease phenotypes.
- Optimization: Osaka University’s single-nick guided DNA (SNGD) technology synchronizes target and donor DNA nicking, reducing error rates from 90% to 5%.
2. Large Deletion & Chromosomal Rearrangement Repair
For diseases caused by large deletions (e.g., DMD), multiplex CRISPR-Cas9 gRNAs induce large-scale deletions or chromosomal rearrangements. Excision of non-essential exons in the DMD gene restores functional protein expression, avoiding unpredictable indels.
II. DSB-Free Precision Editing
1. Base Editing
- Mechanism: Combines Cas9 nickase (nCas9) with deaminases to catalyze base conversions (C→T or A→G). Beam Therapeutics’ A-to-G editor corrected the HBB Glu6Val mutation in sickle cell anemia mice, normalizing red blood cell function.
- Advantage: Avoids DSB-induced genomic instability, ideal for SNP-linked diseases like β-thalassemia.
2. Prime Editing
- Mechanism: Reverse transcriptase fused to nCas9 synthesizes new DNA strands using pegRNA templates, enabling any base edit, insertion, or deletion. Prime editing achieved 70% correction of the CFTR ΔF508 mutation in cystic fibrosis models.
3. Epigenome Editing
- CRISPRi/a: dCas9 fused to epigenetic regulators (e.g., DNMT3A, p300) silences or activates genes. In Huntington’s disease, CRISPRi suppressed mutant HTT transcription, reducing toxic protein aggregation.
III. Delivery Innovations & Clinical Translation
1. Viral & Non-Viral Vectors
- AAV Vectors: Deliver CRISPR components in vivo. AAV-mediated editing of CEP290 mutations in Leber congenital amaurosis is in Phase III trials.
- Lipid Nanoparticles (LNPs): Liver-targeted LNPs encapsulating Cas9 mRNA and gRNA achieved 40% editing efficiency in hereditary ATTR amyloidosis.
2. Stem Cell & Ex Vivo Editing
- iPSC Engineering: Patient-derived iPSCs are edited ex vivo and differentiated into functional cells. In sickle cell anemia, corrected iPSCs generated healthy hematopoietic stem cells, restoring normal erythropoiesis.
IV. Clinical Applications & Breakthroughs
1. Hematologic Diseases
- Sickle Cell Anemia & β-Thalassemia: CRISPR Therapeutics’ CTX001 edits BCL11A to boost fetal hemoglobin, with 90% of trial patients achieving transfusion independence.
- Hemophilia A: HDR repaired F8 intronic inversions, restoring Factor VIII activity in animal models.
2. Inherited Ocular Diseases
- Leber Congenital Amaurosis: Editas Medicine’s EDIT-101 (AAV-delivered CRISPR-Cas9) excises the CEP290 IVS26 mutation, earning FDA Breakthrough Therapy designation.
3. Cancer Therapy
- CAR-T Engineering: CRISPR-knockout of PD-1 enhances T cell antitumor activity. Novartis’ CTX130 showed a 60% objective response rate in lymphoma trials.
V. Challenges & Future Directions
1. Technical Hurdles
- Off-Target Effects: High-fidelity Cas9 variants (e.g., HypaCas9) and AI-guided gRNA design (e.g., DeepCRISPR) reduce off-target rates below 0.1%.
- Delivery Efficiency: Tissue-specific LNPs (e.g., GalNAc-liver targeting) and novel viral vectors (e.g., AAVrh74) are under development.
2. Clinical Barriers
- Immunogenicity: Humanized Cas9 (e.g., SaCas9-KKH) or transient mRNA expression mitigates anti-Cas9 immune responses.
- Ethics & Regulation: Germline editing remains controversial, with current trials limited to somatic cells.
3. Emerging Frontiers
- AI-Driven Design: AlphaFold-predicted protein-DNA interactions optimize editor structures and specificity.
- Quantum Biology Tools: Gold nanoparticle-enhanced Raman spectroscopy enables real-time editing monitoring for dynamic adjustments.
Conclusion
Gene editing has evolved from a lab tool to a transformative clinical force. Through DSB repair, base editing, and epigenome modulation—coupled with delivery system advancements—humans are nearing the vision of “one-time edits for lifelong cures.” With AI, single-cell omics, and synthetic biology, gene editing is advancing toward high-precision, low-risk “molecular surgery,” offering根治 solutions for thousands of genetic disorders. As Jennifer Doudna stated: “We are rewriting life’s source code, with CRISPR as the compiler of this biological revolution.”
Data sourced from publicly available references. For collaborations or domain inquiries, contact: chuanchuan810@gmail.com.