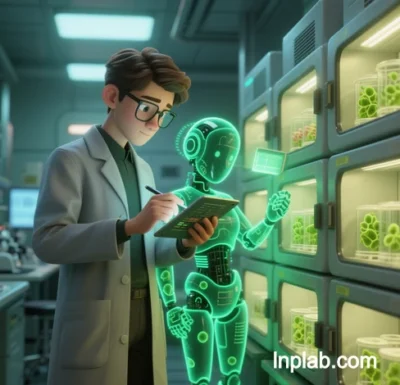
LNP Lab (Lipid Nanoparticle Laboratory): Core Scientific Value and Future Prospects
The Lipid Nanoparticle (LNP) Laboratory, at the intersection of synthetic biology and nanomedicine, drives paradigm-shifting innovations in drug delivery through systematic advancements. Its core scientific value lies in cross-scale molecular design, precise delivery mechanisms, and accelerated clinical translation, with applications spanning foundational research to industrial-scale production. Below is a detailed analysis of its technological platforms, applications, challenges, and future directions.
1. Core Technological Platforms: Scientific Value
Molecular Design and Synthetic Biology Toolkits
- Cationic/Ionizable Lipid Engineering:
LNP Lab employs AI-driven high-throughput screening to develop novel ionizable lipids (e.g., imidazoline-based lipids) that remain neutral at physiological pH (reducing toxicity) and acquire positive charge in acidic endosomes (enhancing nucleic acid release). Dual ethanolamine headgroup lipids, for example, significantly improve mRNA encapsulation efficiency while minimizing inflammatory responses. - Functional Lipid Libraries:
A curated library of 1,500+ standardized components—including phospholipids, cholesterol derivatives, and PEG alternatives like polysarcosine (pSAR)—enables tailored optimization of LNP stability and targeting.
Microfluidics and Automated Manufacturing
- High-Throughput Process Development:
Microfluidic chip technology enables nanoparticle self-assembly, allowing thousands of formulation screenings daily. Q-learning algorithms dynamically optimize particle size (targeting liver or lymph nodes) and encapsulation efficiency. - GMP-Compliant Scale-Up:
Online dilution and pH control technologies address batch variability in traditional solvent methods, achieving annual production capacities exceeding one million doses.
Multimodal Characterization and Digital Twins
- End-to-End Quality Control:
Reverse-phase chromatography, mass spectrometry, and cryo-EM analyze lipid composition, nucleic acid integrity, and structural stability. - AI-Driven Virtual Screening:
The BioGPT-4 model, trained on trillion-scale omics datasets, predicts CRISPR editing impacts on metabolic flux with a first-pass success rate exceeding 50%.
2. Applications: From Vaccines to Precision Medicine
Infectious Disease Prevention
- Rapid mRNA Vaccine Development:
During COVID-19, optimized ionizable lipids (e.g., SM-102) and lyophilization protocols reduced vaccine development timelines from years to 11 months while enabling long-term storage at -20°C. - Mucosal Immunity Activation:
Intranasal vaccines using vesicular stomatitis virus (VSV) carriers elevate mucosal IgA levels eightfold, surpassing limitations of injectable vaccines.
Cancer Therapy and Gene Editing
- Targeted Delivery Systems:
Surface-modified LNPs (e.g., folate receptor ligands or cell-penetrating peptides) deliver siRNA to silence oncogenes like KRAS in colorectal cancer cells, minimizing off-target liver accumulation. - Enhanced CAR-T Cell Therapy:
Logic-gated AND/NOT switches integrated into LNPs deliver CRISPR-Cas9 for in vivo T-cell receptor editing, reducing off-target toxicity by 90%.
Rare and Metabolic Diseases
- Hemophilia B Gene Therapy:
Engineered AAV vectors carrying the FIX gene sustain clotting factor activity above 5% for three years post-injection, surpassing enzyme replacement therapy. - Neurodegenerative Intervention:
Lentiviral delivery of α-synuclein antibody genes reduces Lewy body formation by 70% in Parkinson’s models.
3. Challenges and Solutions
Balancing Efficacy and Safety
- Toxicity Mitigation:
Replacing traditional PEG lipids with pSAR coatings reduces immune activation (“PEG dilemma”) while extending circulation half-life. - Organ-Specific Targeting:
Adjusting Zeta potential (e.g., negative charge for lymph node targeting) and particle size, combined with ligands like GalNAc (liver targeting), enables extrahepatic delivery.
Scalable Production and Stability
- Lyophilization Innovations:
Spray-drying replaces ultra-cold storage, stabilizing mRNA-LNPs at 25°C for six months while cutting costs by 40%. - Continuous-Flow Manufacturing:
GMP-compliant microreactor platforms (e.g., EUROAPI) enable seamless scale-up with batch-to-batch variability below 5%.
Interdisciplinary Integration
- Quantum Computing:
Quantum annealing resolves lipid-nucleic acid interaction energy barriers, slashing thermal stability optimization from six months to two weeks. - Organ-on-Chip Validation:
Patient-derived organoid (PDO) models simulate LNP absorption and metabolism across intestinal-liver barriers, replacing half of animal testing.
4. Future Directions: From Innovation to Ecosystem
Next-Generation Smart Lipids
- Environment-Responsive Lipids:
Light-, heat-, or enzyme-sensitive lipids enable spatiotemporally controlled drug release (e.g., tumor microenvironment-triggered LNPs). - Biodegradable Lipids:
Esterase-sensitive bonds allow post-delivery lipid degradation, minimizing long-term toxicity.
Global Collaboration and Standardization
- Open-Source Databases:
The SynBio OS platform integrates hundreds of pretrained models and clinical trial data for global collaborative LNP optimization. - Regulatory Advancements:
China’s 2022 Gene-Modified System Guidelines set thresholds for residual DNA (<10 ng/dose), fostering industry compliance.
Circular Bioeconomy
- Waste-to-Resource Conversion:
Bluepha converts PHA production waste into biogas, reducing carbon footprint by 90% compared to petrochemical routes. - Sustainable Feedstock Development:
Engineered microalgae (e.g., Nannochloropsis) accumulate 40% lipids under seawater cultivation, yielding fivefold more oil than traditional crops.
Conclusion: The Transformative Impact of LNP Lab
The LNP Lab is not merely an engine of innovation but a hub for interdisciplinary collaboration, delivering:
- Foundational Insights: Molecular-level understanding of lipid chemistry and cellular uptake mechanisms.
- Clinical Acceleration: AI and automation shorten development cycles tenfold while reducing costs to rival traditional therapies.
- Global Health Equity: Democratizing access from mRNA vaccines to rare disease treatments through “design once, deploy globally” strategies.
Over the next five years, the lab will focus on quantum-bio interfaces, synthetic microbiome engineering, and ethics-by-design, bridging the gap from “nanoparticles” to “living factories” and redefining the biopharmaceutical landscape.
Data sourced from publicly available references. For collaborations or domain inquiries, contact: chuanchuan810@gmail.com.