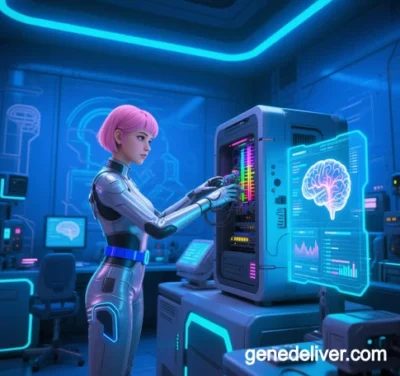
Gene Delivery: A Critical Step in Gene Therapy, Vaccine Development, and Biomedical Research
Gene delivery—the process of introducing genetic material into target cells—is foundational to advancing gene therapies, next-generation vaccines, and biomedical discoveries. Its success hinges on precision, efficiency, and safety across diverse biological systems. Below, we explore the mechanisms, applications, and innovations shaping this field.
I. Mechanisms of Gene Delivery
1. Viral Vectors
- Adeno-associated viruses (AAVs): Engineered for low immunogenicity and tissue specificity (e.g., AAV9 for crossing the blood-brain barrier).
- Lentiviruses: Enable stable gene integration in dividing and non-dividing cells, critical for hematopoietic stem cell therapies.
- Adenoviruses: High transduction efficiency but transient expression, used in COVID-19 vaccines (e.g., AstraZeneca).
2. Non-Viral Vectors
- Lipid nanoparticles (LNPs): Protect mRNA from degradation and facilitate cellular uptake (e.g., Pfizer-BioNTech’s COVID-19 vaccine).
- Electroporation: Electric pulses create transient pores in cell membranes for DNA/RNA delivery, widely used in CAR-T cell engineering.
- CRISPR-Cas9 ribonucleoproteins (RNPs): Direct delivery of Cas9 protein and guide RNA reduces off-target effects.
II. Applications Across Biomedicine
1. Gene Therapy
- Monogenic disorders: AAV-delivered CFTR gene restores chloride channel function in cystic fibrosis.
- Cancer immunotherapy: Lentiviral vectors engineer T cells with chimeric antigen receptors (CARs) for targeted tumor killing.
2. Vaccine Development
- mRNA vaccines: LNPs encapsulate mRNA encoding viral antigens (e.g., spike protein for SARS-CoV-2).
- DNA vaccines: Electroporation enhances plasmid delivery, inducing robust T-cell responses (e.g., Zika virus trials).
3. Biomedical Research
- Gene editing: Viral vectors deliver CRISPR components to organoids for disease modeling (e.g., Alzheimer’s in cerebral organoids).
- Functional genomics: Lentiviral shRNA libraries enable genome-wide knockout screens.
III. Key Challenges
1. Targeting Specificity
- Off-organ toxicity: Systemic AAV administration may lead to liver sequestration (e.g., high-dose AAV9 elevates liver enzymes).
- Capsid engineering: Directed evolution generates tissue-specific AAV variants (e.g., AAV-PHP.eB for CNS targeting).
2. Immune Responses
- Pre-existing immunity: Neutralizing antibodies against viral vectors limit re-dosing. Hybrid LNPs with stealth polymers (e.g., PEGylation) mitigate recognition.
- Innate immune activation: mRNA LNPs with nucleoside modifications reduce TLR-mediated inflammation (e.g., Moderna’s mRNA-1273).
3. Scalability and Cost
- Manufacturing complexity: Viral vector production requires high-quality cell lines and costly purification. Modular platforms (e.g., baculovirus-insect systems) lower costs.
- Storage limitations: Lyophilized LNPs enable thermostable mRNA vaccines for resource-limited regions.
IV. Emerging Innovations
1. Synthetic Biology
- Programmable vectors: CRISPR-based logic gates enable conditional gene expression (e.g., hypoxia-activated promoters in tumor targeting).
- Artificial viruses: De novo-designed capsids with tunable tropism (e.g., machine learning-generated AAV variants).
2. Advanced Delivery Systems
- Exosome-based delivery: Plant-derived exosomes carry siRNA for inflammatory disease treatment with minimal immunogenicity.
- Hydrogel depots: Sustained release of LNPs from biodegradable hydrogels enhances localized gene editing (e.g., diabetic wound repair).
3. AI-Driven Optimization
- Predictive modeling: Deep learning predicts LNP composition for optimal organ targeting (e.g., liver vs. lung).
- Automated screening: High-throughput platforms test thousands of vector formulations for gene delivery efficiency.
V. Future Directions
- In vivo gene editing: LNPs delivering base editors correct mutations without double-strand breaks (e.g., PCSK9 silencing for hypercholesterolemia).
- Ecosystem-scale delivery: Engineered phages transfer genes to environmental microbiomes for bioremediation.
- Ethical frameworks: Regulatory guidelines for germline editing and environmental release of gene-drive organisms.
Data sourced from public references. Contact: chuanchuan810@gmail.com.
Gene Delivery Explained
Gene Delivery refers to the process of introducing exogenous genes into target cells or organisms using specific technologies or vectors, serving as a critical step in gene therapy, vaccine development, and biomedical research
1. Core Concepts
Purpose: To precisely deliver therapeutic genes (e.g., for mutation repair or antigen expression) to target cells.
Vector Types:
Viral Vectors (e.g., adenovirus, lentivirus): Naturally high infection efficiency.
Non-viral Vectors (e.g., liposomes, nanoparticles): Higher safety but lower efficiency.
2. Key Applications
Field Application Examples Technical Features
Gene Therapy Delivering functional genes to replace defective ones (e.g., β-thalassemia) Requires long-term stable expression1
Vaccine Development Delivering viral antigen genes (e.g., Spike protein gene in mRNA vaccines) Rapid response to new pathogens
Agricultural Breeding Introducing pest/drought-resistant genes (e.g., Bt crops)3 Requires overcoming species barriers
3. Technical Challenges
Targeting Specificity: Avoiding off-target gene delivery.
Immune Response: Viral vectors may trigger host rejection.
Expression Control: Precise regulation of gene expression duration and intensity.
Current research focuses on optimizing novel vectors (e.g., AAV) and delivery systems (e.g., CRISPR-Cas9 complexes).