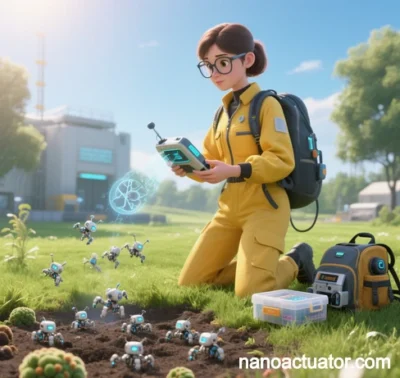
Nanoactuators: Advances in Neural Probes, Brain-Computer Interfaces, and Targeted Drug Delivery
I. Core Principles
Nanoactuators are intelligent devices driven by external energy sources (e.g., light, magnetic fields, electricity, chemical gradients) to achieve nanoscale motion or mechanical deformation. Their applications in neuroscience and drug delivery focus on:
- Dynamic Precision Control: Enabling high spatiotemporal resolution neural signal recording/stimulation or controlled drug release through micro-nanoscale mechanical motion.
- Multimodal Energy Integration: Combining magnetic, optical, and ultrasonic inputs to penetrate biological barriers (e.g., blood-brain barrier) and enhance tissue targeting.
- Adaptive Feedback Mechanisms: Integrating sensing and actuation to dynamically adjust operations in response to physiological signals (e.g., pH, oxidative stress).
II. Breakthroughs in Neural Probes and Brain-Computer Interfaces
1. Innovations and Case Studies
- Flexible Nanoelectrode Arrays:
- Magnetic-Optical Dual-Driven Probes: Ultrathin silicon nitride substrates embedded with magnetic nanoparticles and gold nanorods use alternating magnetic fields for brain penetration, while near-infrared light triggers localized deformation to enhance neuron-electrode contact.
- Piezoelectric Nanowire Arrays: Zinc oxide nanowires generate piezoelectric potentials from mechanical deformation, enabling wireless recording of cortical neuron activity and ultrasound-triggered calcium release for synaptic modulation.
- Closed-Loop Brain-Computer Interfaces (BCIs):
- Optogenetic-Nanoactuator Hybrids: Graphene quantum dot-coated nanoactuators with light-sensitive channel proteins (ChR2) decode motor intent in paralyzed patients, enabling low-latency robotic arm control.
2. Advantages and Challenges
- Advantages:
- Minimally Invasive: Nanoscale size reduces tissue damage and glial scarring.
- High Resolution: Optical actuators achieve single-neuron targeting precision.
- Challenges:
- Material Stability: Biodegradation in biological fluids necessitates bioinspired coatings (e.g., polydopamine encapsulation).
- Energy Efficiency: Improving energy conversion rates for multimodal systems.
III. Innovations in Targeted Drug Delivery
1. Blood-Brain Barrier (BBB) Penetration
- Magnetic-Ultrasound Synergy: Iron oxide nanoactuators guided by magnetic fields use low-frequency ultrasound to transiently open BBB tight junctions, enhancing drug delivery to brain tumors.
- Bioinspired Leukocyte Mimetics: CD11b antibody-decorated nanoactuators mimic white blood cell migration to cross BBB endothelial cells, delivering siRNA to Alzheimer’s plaque regions.
2. Disease-Responsive Release
- Redox-Sensitive Systems: Cadmium selenide nanoactuators release Parkinson’s drugs in high oxidative stress environments via glutathione-triggered disulfide bond cleavage.
- Light-Controlled Sequential Delivery: Upconversion nanoparticles absorb near-infrared light to release anti-inflammatory drugs and neurotrophic factors sequentially, promoting post-stroke neural repair.
3. Optimization Strategies
- Targeting Precision: Dual-ligand modifications (e.g., Angiopep-2 + TfR antibodies) improve brain tumor delivery efficiency.
- Safety Enhancements: Low-intensity focused ultrasound with microbubbles enables non-invasive, precise actuation.
IV. Cross-Disciplinary Integration and Future Trends
1. Neurorepair-Drug Delivery Integration
- Self-Powered Systems: Piezoelectric polymer (PVDF) nanoactuators harness cerebrospinal fluid flow to stimulate nerve growth and release neurotrophic factors, aiding spinal cord injury recovery.
2. AI-Enhanced Nanoactuators
- Dynamic Path Planning: Reinforcement learning algorithms optimize magnetic nanoactuator navigation in real time, doubling delivery speed within brain tissue.
- Digital Twin Platforms: MRI-based vascular network simulations predict nanoactuator behavior and drug release kinetics, improving clinical trial success rates.
3. Clinical Translation Challenges
- Manufacturing Scalability: Microfluidic synthesis reduces batch variability.
- Ethical Safeguards: Implementing fail-safe mechanisms (e.g., pH-sensitive self-destruction) for autonomous systems.
V. Conclusion and Outlook
Nanoactuators are transforming neuroscience and drug delivery from static interventions to dynamic, adaptive therapies:
- Neural Interfaces: Subcellular-precision BCIs may restore motor function in paralysis by 2030.
- Drug Delivery: Multimodal systems could elevate brain drug concentrations to therapeutic thresholds for Alzheimer’s and other CNS diseases.
Future integration with synthetic biology and quantum sensing may enable “nano-surgeons” capable of single-neuron repair and molecular network regulation.
Data sourced from public references. For collaboration or domain inquiries, contact: chuanchuan810@gmail.com