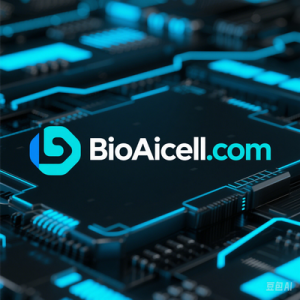
: An In-Depth Analysis
Biocyclic is a compound term combining Bio (biological) and Cyclic (circular), typically referring to “biological cycles” or “biologically periodic systems.” Its meaning varies across disciplines, with applications in ecology, agriculture, materials science, and more. Below is a comprehensive breakdown of its definitions, principles, and real-world implementations:
I. Core Definitions and Multidisciplinary Applications
- Ecology and Sustainability:
- Biocyclic System: A natural or engineered ecosystem where materials (e.g., carbon, nitrogen, phosphorus) and energy flow in closed loops through biological processes (decomposition, metabolism, regeneration), minimizing reliance on external resources.
- Applications: Urban organic waste composting, wetland purification systems, nutrient cycling in forest ecosystems.
- Agriculture and Organic Production:
- Biocyclic Agriculture: A self-sufficient organic farming model that eliminates external inputs by relying on plant residues, green manure, and microbial activity to maintain soil fertility.
- Case Studies:
- Biocyclic Vegan Agriculture: Avoids animal-derived fertilizers (e.g., manure), using plant-based compost and biochar (e.g., Greece’s Biocyclic Vegan Network).
- Closed-Loop Farms: German farms achieving energy and nutrient autonomy via crop rotation and anaerobic digestion systems.
- Materials Science and Industry:
- Biocyclic Materials: Fully degradable or biologically regenerated materials like bioplastics (PLA, PHA) and mycelium-based packaging.
- Goal: Replace petroleum-based materials, reduce microplastic pollution, and advance “Cradle-to-Cradle” design principles.
II. Technical Principles and Standards
- Key Mechanisms of Ecological Cycling:
- Decomposer-Driven Processes: Fungi and bacteria break down organic matter into plant-accessible nutrients.
- Symbiotic Relationships: Collaboration between plants and rhizosphere microbes (e.g., mycorrhizal fungi) enhances nutrient efficiency.
- Energy Flow: Solar energy converted into biomass via photosynthesis sustains all life within the system.
- Biocyclic Standards:
- Biocyclic Vegan Certification:
- Requirements: Prohibits animal-derived inputs (e.g., fishmeal, bone meal); relies on plant compost and biodiversity management.
- Scope: Organic farms in the EU, Japan, South Africa, and beyond.
- ISO Standards for Biocyclic Materials (e.g., ISO 16620): Govern biodegradability, carbon footprint, and circular performance of bio-based materials.
III. Industry Case Studies
- Agriculture:
- Biocyclic Olive Groves (Greece): Use leguminous cover crops (e.g., alfalfa) for nitrogen fixation and olive residue compost, reducing synthetic fertilizer use by 90%.
- Biocyclic Rice Farming (Japan): Combines rice husk biochar with duck-integrated weed control for zero-pesticide production.
- Materials Innovation:
- Mycelium Packaging (Netherlands): Mushroom-root-based materials replace polystyrene foam, degrading naturally in 28 days.
- PHA Bioplastics (USA): Microbially synthesized and fully marine-degradable.
- Urban Ecology:
- Prinzessinnengarten (Berlin): A community farm using food waste compost and rainwater harvesting to create a closed-loop food-energy-water system.
IV. Challenges and Future Directions
- Technical Barriers:
- Efficiency: High energy demands for producing biodegradable materials (e.g., PLA requiring high-temperature composting).
- Scalability Costs: Initial investment for closed-loop farming systems is 2-3x higher than conventional agriculture.
- Standardization: Lack of a unified global certification framework for Biocyclic practices.
- Innovation Opportunities:
- Synthetic Biology: Engineer microbes (e.g., Ideonella sakaiensis for plastic degradation) to enhance pollutant breakdown.
- AI Optimization: Machine learning to predict optimal composting formulas or crop rotation patterns.
- Policy Incentives: Carbon taxes or subsidies to encourage Biocyclic adoption.
V. Ethical and Social Considerations
- Ecological Risks:
- Engineered microbes could disrupt natural ecosystems through gene transfer.
- Vegan agriculture risks soil degradation without careful nitrogen management.
- Equity Concerns:
- High certification costs may exclude small-scale farmers from Biocyclic supply chains.
Conclusion
Biocyclic embodies a paradigm that merges biological principles with circular economy practices, aiming to mimic nature’s self-sustaining systems and reduce humanity’s linear resource consumption. Despite technical and economic hurdles, its applications in agriculture, materials, and urban planning demonstrate transformative potential, positioning it as a key strategy against climate change and resource depletion.
If you are interested in purchasing this domain, please contact: chuanchuan810@gmail.com