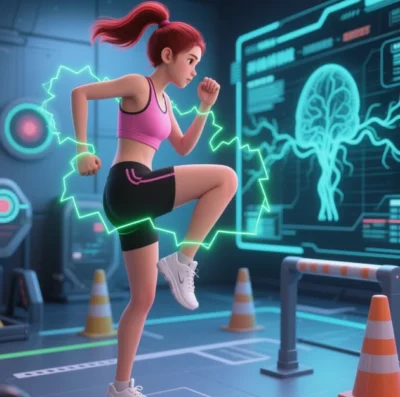
Haptisense and Neurobiological Mechanisms: A Multidimensional Analysis
Haptisense (haptic perception) represents the integration of tactile technology with neurobiology, aiming to simulate or enhance the neural encoding and decoding mechanisms of human touch. This analysis explores its foundations across five dimensions: neural signaling, brain region mapping, multisensory integration, applications, and future challenges.
1. Neurobiological Basis of Haptic Perception
Peripheral Neural Encoding
- Mechanoreceptor Specialization:
- Meissner corpuscles detect dynamic touch (e.g., slippage), Merkel cells sense static pressure, Pacinian corpuscles respond to high-frequency vibrations, and Ruffini endings monitor skin stretch. Signals travel via myelinated Aβ fibers to the spinal cord.
- Temperature and Pain Pathways: Unmyelinated C fibers transmit thermal and nociceptive signals to the thalamus (VPL nucleus) and primary somatosensory cortex (S1).
Central Nervous System Processing
- Thalamocortical Pathways: The thalamus relays tactile signals to S1, while the parietal association cortex (e.g., Brodmann area 5) enables spatial localization and object recognition. The prefrontal cortex (PFC) and insula evaluate emotional valence (e.g., pleasure or discomfort).
- Sensorimotor Integration: The cerebellum adjusts motor commands by comparing expected and actual tactile feedback, refining grip precision.
2. Neural Mechanism Simulation in Haptisense
Biomimetic Tactile Feedback
- Vibration Modulation: Adjusting amplitude and frequency mimics textures (e.g., high-frequency vibrations activate Pacinian corpuscles).
- Example: Sony’s DualSense controller uses electromagnetic actuators to simulate surface hardness, activating force-sensitive neurons in S1.
- Cross-Modal Cortical Activation:
- Functional MRI shows that virtual touch (e.g., interacting with digital avatars) elicits PFC and insula activation patterns resembling real-world touch, driven by visuo-tensory integration.
- Real-time prediction algorithms (e.g., Kalman filters) minimize latency between visual and tactile feedback, preserving immersion.
3. Multisensory Integration in Neurobiology
Visuo-Haptic Cortical Synergy
- Dorsal Pathway Integration: Visual motion (MT area) and tactile spatial data (VIP area) collaborate for 3D object manipulation. VR gloves reduce visual weight estimation errors by enhancing haptic feedback.
- Mirror Neuron Activation: Observing others’ tactile actions triggers mirror neurons in the inferior frontal gyrus (IFG), enabling empathy through simulated social touch (e.g., virtual handshakes).
Audio-Tactile Phase Locking
- Cross-Modal Rhythm Coupling: Synchronizing tactile vibrations with auditory beats enhances theta-wave coherence between auditory (A1) and somatosensory (S1) cortices, improving immersion in rhythm games.
4. Medical Neuroengineering Applications
Neural Prosthetics
- Cortical Microstimulation (ICMS): Microelectrode arrays in S1 simulate pressure gradients, enabling amputees to perceive grip force levels.
- Peripheral Nerve Interfaces: Direct stimulation of residual nerves (e.g., median nerve) restores thermal and texture perception via spatiotemporal encoding.
Surgical Robotics
- Force-Position Hybrid Control: Systems like the Da Vinci Surgical System reduce operative errors by providing tissue elasticity feedback, enhancing parietal cortex representation of tool-tissue interactions.
- Pathological Tactile Databases: Machine learning models correlate tumor stiffness with malignancy, guiding real-time haptic alerts during procedures.
5. Challenges and Future Directions
Biological Limits of Neural Decoding
- Bandwidth Constraints: Current haptic interfaces transmit data at speeds far below natural tactile systems. Quantum sensors and optogenetics may overcome this.
- Affective Touch Encoding: Engineering emotionally nuanced signals (e.g., CT-mediated caresses) remains challenging due to limbic system complexity.
Interdisciplinary Convergence
- Bidirectional Brain-Computer Interfaces (BCIs): Merging haptic feedback with motor intent decoding could enable seamless “perception-action” loops.
- Synthetic Neuromorphic Devices: Memristor arrays replicating adaptive receptor firing could yield low-power, biomimetic haptic chips.
Conclusion
Haptisense technology computationally replicates neurobiological mechanisms, driven by systematic deconstruction of molecular, circuit, and behavioral layers of tactile pathways. Advances in optogenetics and neuromorphic computing may transition haptic engineering from “simulated feedback” to “neural fusion,” bridging biological and digital worlds.
Data sourced from public references. For collaboration or domain inquiries, contact: chuanchuan810@gmail.com