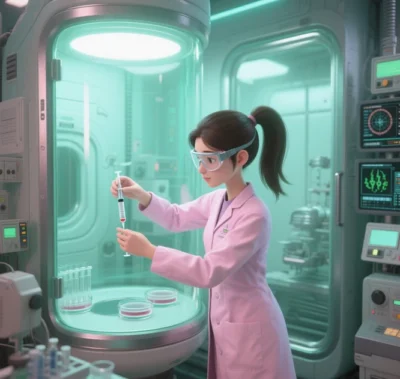
Modified RNA in Therapeutics and Vaccines: Mechanisms, Applications, and Future Challenges
Modified RNA technology enhances RNA stability, translation efficiency, and immune regulation through chemical or sequence engineering, making it a cornerstone of modern drug and vaccine development. Below, we analyze its mechanisms, therapeutic applications, vaccine advancements, and future challenges.
I. Core Mechanisms of Modified RNA
- Chemical Modifications to Reduce Immunogenicity
- Nucleotide Substitution: Replacing uridine with pseudouridine (Ψ), N1-methylpseudouridine (m1Ψ), or 5-methylcytosine (m5C) avoids recognition by innate immune receptors (e.g., TLR3/7/8, RIG-I), minimizing type I interferon responses while boosting mRNA stability and translation.
- Cap Structure Optimization: Anti-reverse cap analogs (ARCA) or Cap1 structures enhance ribosome binding and prolong mRNA half-life.
- Delivery System Innovations
- Lipid Nanoparticles (LNPs): Ionizable lipids, phospholipids, and PEG protect mRNA from nucleases and promote endosomal escape. LNP composition can modulate immune activation (e.g., adjuvant lipids).
- Non-Viral Vectors: Polymer nanoparticles (e.g., PEI), exosomes, or virus-like particles (VLPs) enable tissue- or cell-specific targeting (e.g., dendritic cells).
- Sequence Engineering for Enhanced Function
- Codon Optimization: Adjusting G:C content and codon usage frequency improves translation efficiency (e.g., Moderna’s spike protein expression increased fourfold).
- Self-Amplifying RNA (saRNA): Incorporates alphavirus replicase genes for intracellular RNA replication, reducing dose requirements (e.g., Arcturus’s ARCT-154 vaccine uses one-tenth the dose of traditional mRNA).
II. Therapeutic Applications: From Rare Diseases to Cancer Immunotherapy
- Rare Diseases and Metabolic Disorders
- Protein Replacement Therapy:
- Propionic Acidemia: Moderna’s mRNA-3927 encodes propionyl-CoA carboxylase, delivered via LNPs to hepatocytes to restore enzyme activity (Phase I/II trials).
- Glycogen Storage Disease Type 1a (GSD1a): mRNA-3745 encodes glucose-6-phosphatase to correct hepatic metabolic defects.
- Cancer Immunotherapy
- Personalized Neoantigen Vaccines:
- Melanoma: BioNTech’s mRNA-4157 encodes patient-specific neoantigens (20–34 targets), activating CD8+ T cells. Combined with PD-1 inhibitors, it improves 2-year survival to 62%.
- Universal Vaccine Chassis: CAR-NK 3.0 cells with synthetic RNA modules enable dynamic neoantigen updates for long-term protection.
- Immune Modulator Delivery: mRNA-encoded cytokines (e.g., IL-12, IFN-α) or co-stimulatory molecules (e.g., CD40L) remodel the tumor microenvironment.
- Genome Editing and Gene Therapy
- CRISPR-Cas9 Systems: mRNA encodes Cas9 and sgRNA for transient expression, reducing off-target risks (e.g., Intellia’s NTLA-2001 targets transthyretin amyloidosis via LNPs).
- Base/Epigenome Editing: Modified RNA pairs with dCas9-methyltransferase fusions for precise epigenome editing.
III. Vaccine Development: From Infectious Diseases to Universal Designs
- Infectious Disease Vaccines
- Rapid Response Platforms:
- COVID-19: Moderna’s mRNA-1273 and BioNTech’s BNT162b2 use m1Ψ-modified RNA, enabling redesign within 72 hours of variant emergence (95% efficacy).
- Influenza and RSV: Moderna’s mRNA-1010 (seasonal flu) and mRNA-1345 (RSV) cover multiple subtypes with 75% cross-protection in Phase III trials.
- Self-Amplifying RNA Vaccines: ARCT-154 for SARS-CoV-2 variants induces eightfold higher neutralizing antibodies with a single dose (approved in Japan).
- Preventive Cancer Vaccines
- HPV-Associated Cancers: mRNA encoding HPV E6/E7 proteins induces neutralizing antibodies and T-cell responses to prevent cervical and head/neck cancers.
- Universal Tumor Antigens: Telomerase (hTERT) or carcinoembryonic antigen (CEA) combined with adjuvant RNA (e.g., CpG motifs) enhances immunogenicity.
- Immune Tolerance Induction
- Autoimmune Diseases: Modified RNA encoding self-antigens (e.g., insulin, myelin) induces regulatory T cells (Tregs) to treat type 1 diabetes and multiple sclerosis.
IV. Challenges and Future Directions
- Technical Bottlenecks
- Delivery Precision: Current LNPs primarily target hepatocytes; tissue-specific carriers (e.g., lung-targeted lipids) are needed.
- Controlled Expression: Self-destructing RNA (e.g., miRNA-responsive elements) or temperature-sensitive switches enable on-demand protein expression.
- Industrialization Barriers
- Cost and Accessibility: TdT enzyme-driven DNA synthesis reduces costs, while decentralized microfluidic factories cut production cycles to two weeks.
- Cold Chain Independence: Lyophilized formulations (e.g., CureVac’s CVnCoV) and stabilized LNPs enable six-month storage at 2–8°C.
- Ethical and Regulatory Hurdles
- Dynamic Risk Assessment: AI models monitor off-target effects and immunotoxicity; FDA’s “rolling review” accelerates approvals.
- Biosafety Standards: Synthetic biology frameworks (e.g., ISO/TC 276) regulate artificial genome and chassis cell design.
V. Future Outlook: Transforming Healthcare Paradigms
- Hyper-Personalized Medicine: Single-cell multi-omics (epigenomics + metabolomics) drive nanovaccine design for immune subsets (e.g., rejuvenating exhausted T cells).
- AI-Wet Lab Integration: Robotic labs (e.g., DeepSeek) automate design-synthesis-validation cycles for on-demand RNA therapeutics.
- Global Health Equity: Modular RNA factories in developing nations reduce vaccine costs to under $1 per dose.
Data sourced from public references. For collaboration or domain inquiries, contact: chuanchuan810@gmail.com