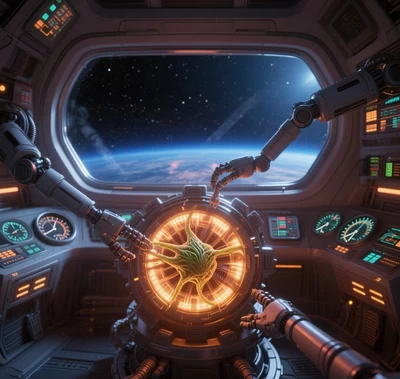
LNP Engine in Biomedicine and Vaccine Development: Core Roles and Innovations
Lipid nanoparticles (LNPs), as a “delivery engine” for nucleic acids, have become a cornerstone technology in gene therapy, vaccine development, and cancer immunotherapy due to their efficiency, flexibility, and engineering adaptability. Below, we analyze their mechanisms, applications, innovations, challenges, and future directions.
I. Technical Mechanisms: Delivery Logic and Optimization
- Structure and Delivery Principles
LNPs are composed of ionizable lipids, helper lipids (e.g., phospholipids), cholesterol, and PEG-lipids, self-assembled into 80–200 nm particles via microfluidic mixing. Core mechanisms include:- Endosomal Escape: Ionizable lipids (e.g., SM-102, ALC-0315) protonate in acidic endosomal environments, triggering lipid membrane phase changes to release nucleic acids into the cytoplasm.
- Targeted Modification: Surface conjugation of antibodies (e.g., anti-EGFR) or ligands (e.g., folate) redirects LNPs to non-liver tissues (e.g., lungs, brain), reducing hepatic first-pass effects.
- Delivery Efficiency Enhancements
- SORT Technology: Adding cationic lipids (e.g., 18:1 SORT) shifts organ targeting (e.g., from liver to spleen or lungs).
- AI-Driven Design: Machine learning models predict lipid combinations’ in vivo behavior, accelerating novel LNP development (e.g., AexeRNA’s AexLNP platform).
II. Applications: From Vaccines to Precision Medicine
- mRNA Vaccine Development
- COVID-19 Vaccines: BNT162b2 (Pfizer/BioNTech) and mRNA-1273 (Moderna) demonstrated LNP efficacy with >95% protection and rapid adaptability to variants.
- Cancer Vaccines: BioNTech’s mRNA-4157 delivers neoantigen-encoding mRNA via LNPs, boosting melanoma patients’ 2-year survival to 62% when combined with PD-1 inhibitors.
- Gene Therapy and Editing
- Rare Disease Treatment: Intellia’s NTLA-2001 (CRISPR-LNP) silences transthyretin (TTR) genes in hepatocytes with a single dose for hereditary amyloidosis.
- In Vivo CAR-T: LNPs deliver mRNA-encoded CAR receptors to reprogram patient T cells directly, bypassing ex vivo expansion (e.g., CD19-CAR-T for leukemia).
- Precision Oncology
- siRNA Delivery: HER2- or KRAS-targeting siRNA-LNPs silence oncogenic pathways, synergizing with chemotherapeutics (e.g., doxorubicin liposomes).
- Immune Microenvironment Modulation: Intratumoral mRNA-LNPs encoding IL-12 or IFN-α reverse immunosuppression, enhancing checkpoint inhibitor responses.
III. Innovations and Breakthroughs
- Lipid Engineering
- Biodegradable Lipids: Ester bond integration (e.g., DLin-MC3-DMA derivatives) reduces toxicity and improves biocompatibility.
- Immunomodulatory Lipids: Cholesterol-rich LNPs enhance immune activation, while β-sitosterol incorporation reduces inflammation for tolerance-inducing vaccines.
- Smart Delivery Systems
- Stimuli-Responsive LNPs: pH-sensitive lipids (e.g., Dlin-KC2-DMA) release payloads in tumor microenvironments; photothermal LNPs enable near-infrared-triggered release.
- Theranostic Integration: LNPs combine fluorescent tags (e.g., quantum dots) with drug delivery for real-time tumor monitoring and treatment.
- Manufacturing Advancements
- Continuous-Flow Microfluidics: T-mixers enable GMP-scale production (60–80 mL/min flow rates), reducing costs.
- Lyophilization: CureVac’s CVnCoV vaccine achieves long-term stability at 2–8°C, eliminating cold-chain dependency.
IV. Challenges and Solutions
- Delivery Limitations
- Hepatic First-Pass Effect: Only 10–20% of LNPs reach target tissues. Solution: SORT technology or antibody conjugation for lung/brain targeting.
- Low Endosomal Escape: Optimize ionizable lipid hydrophobicity (e.g., MC3 lipids) to enhance membrane fusion.
- Safety Concerns
- PEG Immunogenicity: Allergic reactions in some patients. Trend: PEG-free formulations (e.g., Arcturus’ LUNAR platform).
- Inflammatory Side Effects: SM-102 lipids activate IL-1 pathways, causing injection-site pain. Solution: Anti-inflammatory lipid designs (e.g., cholesterol optimization).
- Industrialization Barriers
- Cost and Scalability: Distributed microfluidic factories (e.g., modular African production lines) reduce per-dose costs.
- Regulatory Harmonization: FDA’s “rolling review” accelerates LNP updates for emerging pathogens.
V. Future Directions: From Innovation to Equity
- Multimodal Platforms
- Gene Editing-Immunotherapy Synergy: CRISPR-LNPs combined with CAR-T therapies target genetic-immunologic diseases (e.g., sickle cell anemia and cancer resistance).
- Environmental Monitoring: LNPs detect antibiotic resistance genes or microplastic-related markers in water systems.
- Global Health Equity
- Modular Production Networks: Regional LNP factories (e.g., sub-Saharan Africa) enable localized vaccine production, closing resource gaps.
- Heat-Stable Formulations: Thermotolerant LNPs (e.g., NanoVation Therapeutics) withstand 40°C storage for tropical regions.
- Cross-Disciplinary Fusion
- Quantum Computing: Quantum annealing optimizes multi-gene editing for complex challenges like breast cancer resistance.
- Synthetic Biology: LNPs deliver synthetic gene circuits to dynamically regulate metabolic diseases (e.g., diabetes).
Conclusion
The LNP engine, with its modular design, efficient delivery, and rapid adaptability, has emerged as infrastructure-level technology in biomedicine. From COVID-19 vaccines to CRISPR breakthroughs, its impact continues to expand. With advances in biodegradable lipids, AI-driven design, and targeted delivery, LNPs are poised to transition from broad-spectrum treatments to cell-level precision interventions by 2030, redefining the future of disease therapy.
Data sourced from public references. For collaboration or domain inquiries, contact: chuanchuan810@gmail.com