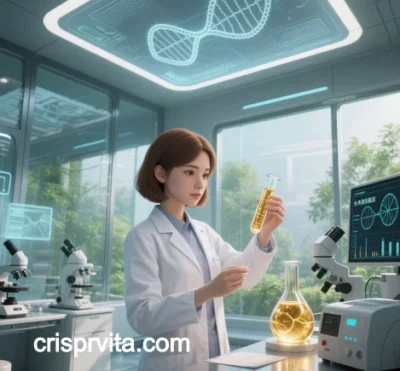
CRISPR-Vita: Applications in Longevity and Quality of Life
CRISPR-Vita (a conceptual platform for CRISPR-based anti-aging interventions) represents a revolutionary advancement in gene editing for extending healthspan and improving life quality. By targeting aging-related genes, eliminating pathological cells, and repairing tissue damage, it offers systemic approaches to combat aging. Below are its key applications and scientific foundations:
I. Targeting “Longevity Genes” and Epigenetic Reprogramming
- Telomere Lengthening:
CRISPR-Vita activates telomerase (TERT) to restore shortened telomeres, delaying cellular senescence. Studies show telomerase reactivation extends lifespan in model organisms.
Advantage: CRISPR provides sustained telomere maintenance compared to small-molecule drugs. - Epigenetic Clock Reset:
Editing regulators like SIRT6 and DNMT3A reverses age-related DNA methylation. SIRT6 overexpression, for example, improves metabolic health and longevity.
Innovation: Single-cell sequencing enables personalized epigenetic reprogramming. - Correcting Progeria Mutations:
Precise repair of LMNA mutations restores nuclear stability in progeria models, extending lifespan.
II. Senescent Cell Clearance and Immune Modulation
- Senolysis:
Knocking out anti-apoptotic genes (e.g., BCL-2) triggers selective death of senescent cells, reducing their burden and improving organ function in preclinical models.
Delivery: Lipid nanoparticles (LNPs) target senescent cell markers (e.g., p16INK4a) for specificity. - SASP Regulation:
Editing macrophages to suppress NF-κB/mTOR pathways reduces pro-inflammatory cytokine release (e.g., IL-6, TNF-α).
III. Direct Intervention in Age-Related Diseases
- Neurodegenerative Disorders:
CRISPR-Vita edits APOE4 or Tau phosphorylation sites to reduce amyloid plaques in Alzheimer’s models. Huntington’s disease therapies in Phase I trials show reduced mutant protein levels. - Cardiovascular Regeneration:
Activating YAP/TAZ pathways in cardiomyocytes enhances heart repair post-infarction, reducing scar tissue and improving function. - Metabolic Disease Reversal:
Targeting liver genes (e.g., FGF21) restores insulin sensitivity in diabetic primates.
IV. Tissue Regeneration and Stem Cell Engineering
- iPSC Optimization:
Editing telomerase and mitochondrial genes (e.g., PGC-1α) boosts iPSC differentiation efficiency and survival, increasing dopamine neuron yield in Parkinson’s models. - Organ-Specific Regeneration:
COL17A1 editing rejuvenates skin by promoting keratinocyte proliferation.
V. Personalized Anti-Aging Therapies
- Genome-Phenome Analysis:
CRISPR-Vita uses genome-wide screening (e.g., DepMap) to predict aging risks and design tailored edits, such as enhancing antioxidant pathways in FOXO3 variant carriers. - Early Intervention:
Liquid biopsy integration enables CRISPR-based clearance of early aging markers (e.g., ctDNA).
VI. Challenges and Ethical Considerations
- Safety:
High-fidelity Cas9 variants (e.g., HypaCas9) reduce off-target effects, while LNP/mRNA delivery minimizes immune responses. - Equity and Ethics:
Global frameworks are needed to ensure equitable access and address societal impacts of lifespan extension.
VII. Future Directions
- Epigenome Editing:
dCas9-EZH2/p300 fusion proteins enable precise chromatin modification without DNA breaks. - Mitochondrial Editing:
TALEN or mtCas9 systems target mitochondrial DNA mutations to combat metabolic aging. - Clinical Roadmap:
- 2025–2030: Cure monogenic aging disorders (e.g., progeria).
- 2030–2040: Multi-gene editing for systemic aging intervention.
- Post-2040: AI-driven “lifespan health management” platforms.
Conclusion
CRISPR-Vita shifts anti-aging research from symptom delay to mechanistic reversal. By integrating gene editing, cell therapy, and big data, it redefines “healthy aging” and could extend human lifespan beyond current limits. Ethical frameworks and global collaboration are critical to ensure equitable benefits.
Data sourced from public references. For collaboration or domain inquiries, contact: chuanchuan810@gmail.com