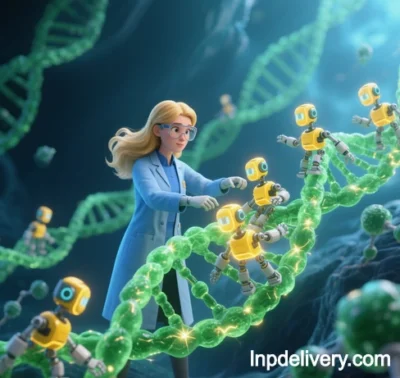
LNP-Delivery: Advances and Prospects in Drug Delivery Technology
Lipid nanoparticles (LNPs) have emerged as a revolutionary drug delivery platform, demonstrating immense potential in RNA therapies, gene editing, and vaccine development. Their core strengths lie in efficiently encapsulating nucleic acid drugs (e.g., mRNA, siRNA), protecting them from degradation, and enabling targeted delivery and controlled release through optimized lipid compositions. Below is a systematic analysis of LNP advancements across four dimensions: technical breakthroughs, applications, challenges, and future trends.
I. Technical Breakthroughs: From Design to Industrialization
- Lipid Composition and Structural Optimization
- Ionizable Lipids: pH-sensitive lipids enhance nucleic acid release in acidic endosomal environments. For example, DLin-MC3-DMA lipids significantly improved cellular uptake efficiency in COVID-19 mRNA vaccines.
- Functional Modifications: Conjugating targeting ligands (e.g., antibodies, peptides) to PEGylated lipids enhances tissue-specific affinity. WuXi STA’s LNP conjugation technology enables precise siRNA delivery to extrahepatic tissues (e.g., tumor microenvironments).
- Manufacturing Innovations
- Microfluidic Mixing: Precision control of flow rates and mixing ratios produces uniform LNPs (<100 nm) with <5% batch variability, enabling clinical-scale production.
- Continuous Processing: DIANT Pharma’s turbulent jet mixing systems integrate real-time analytics to reduce costs and improve batch consistency.
- Stability and Storage Solutions
- Lyophilization: Optimized cryoprotectants (e.g., trehalose, mannitol) extend LNP stability to 12 months at room temperature, bypassing cold-chain logistics.
- Oxidation Resistance: Antioxidant lipids (e.g., vitamin E derivatives) suppress lipid peroxidation, minimizing particle aggregation during storage.
II. Applications: From Vaccines to Precision Therapies
- Infectious Disease Vaccines
- mRNA-LNP Vaccines: COVID-19 vaccines (e.g., Pfizer/BioNTech’s BNT162b2) validated LNP delivery, achieving >10x higher neutralizing antibody titers than traditional vaccines.
- Broad-Spectrum Antiviral Platforms: mRNA-LNP vaccines for influenza and HIV are in Phase II trials, leveraging multivalent antigen designs for cross-protection.
- Cancer Therapeutics
- siRNA-LNP Therapies: Alnylam’s Patisiran uses LNPs to deliver siRNA, silencing disease-causing genes with >80% clinical response rates in hereditary transthyretin amyloidosis.
- CRISPR-LNP Delivery: Intellia Therapeutics’ NTLA-2001 employs LNPs for in vivo CRISPR-Cas9 delivery, sustaining therapeutic effects for over 1 year post-injection.
- Chronic and Genetic Diseases
- Liver-Targeted Delivery: LNPs naturally accumulate in the liver, enabling mRNA therapies for rare liver diseases (e.g., α1-antitrypsin deficiency).
- Beyond the Liver: Surface modifications (e.g., transferrin receptor antibodies) allow LNPs to cross the blood-brain barrier for neurodegenerative disease treatments (e.g., Huntington’s disease).
III. Challenges: Technical and Translational Hurdles
- Immunogenicity and Safety
- Anti-PEG Antibodies: Pre-existing anti-PEG antibodies in ~30% of the population may trigger allergic reactions. Solutions include non-PEG stabilizers (e.g., polysarcosine).
- Endosomal Escape: Only ~5% of LNPs release nucleic acids into the cytoplasm, necessitating lipid phase transition optimization for enhanced membrane fusion.
- Targeting and Efficiency
- Extrahepatic Limitations: Native LNPs primarily target hepatocytes; ligand engineering or charge modulation is needed for lung, spleen, or tumor delivery.
- Tumor Penetration: High interstitial pressure in solid tumors limits LNP diffusion. Ultrasound microbubbles or enzyme-responsive lipids may improve penetration.
- Scalability and Cost
- Raw Material Dependence: Cationic lipid reliance on single suppliers weakens supply chains. CRDMOs are advancing localized production.
- Purification Complexity: Ultrafiltration/chromatography steps account for 40% of costs. Continuous-flow purification could reduce time and resource use.
IV. Future Prospects: Innovation and Trends
- Next-Generation LNP Design
- Modular Platforms: “Plug-and-play” lipid libraries will enable rapid customization of targeting, release kinetics, and immune modulation.
- Biomimetic LNPs: Incorporating cell membrane proteins (e.g., erythrocyte membranes) may enhance immune evasion and circulation time.
- Non-Hepatic Targeting
- Organ-Specific Lipids: AI models predicting lipid-receptor interactions could yield lung-targeted (cystic fibrosis) or heart-targeted (heart failure) LNPs.
- Exosome-LNP Hybrids: Merging exosomes’ homing capabilities with LNP drug-loading capacity may boost tumor specificity.
- Combination Therapies
- LNP-Immune Checkpoint Inhibitors: Co-delivering mRNA-encoded PD-1 antibodies with chemotherapeutics may synergize antitumor immunity.
- Gene-Editing Synergy: Delivering CRISPR-Cas9 with kinase inhibitors via LNPs could simultaneously repair mutations and suppress resistance pathways.
- Regulatory and Global Access
- Standardization: FDA and EMA collaboration on LNP guidelines (e.g., USP <1040>) will streamline global clinical submissions.
- Low-Cost Solutions: Paper-based microfluidics and 3D-printed dispensing devices could democratize LNP access in low-resource regions.
Conclusion
LNP delivery technology is evolving from a “liver-targeted tool” to a “multi-organ precision platform,” overcoming biological barriers and stability limitations of traditional systems. Despite challenges in targeting, immunogenicity, and cost, breakthroughs in AI-driven lipid design, continuous manufacturing, and biomimetics position LNPs as universal solutions for gene therapy, oncology, and chronic disease management within 5–10 years. Ethical frameworks must evolve alongside technological advances to ensure equitable global access to these innovations.
Data sourced from public references. For collaboration or domain inquiries, contact: chuanchuan810@gmail.com