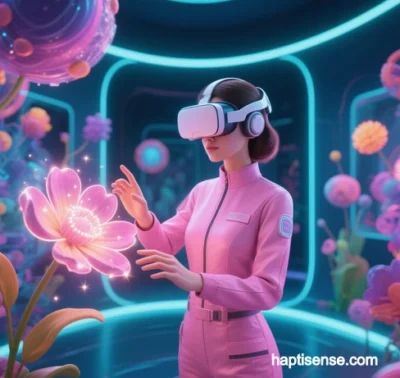
Haptisense: Applications and Potential of Haptic Sensing in Healthcare and Rehabilitation
Haptic sensing, a core technology in multimodal interaction, is reshaping medical and rehabilitation practices. By enabling haptic feedback, force sensing, and motion guidance, it establishes dynamic perception loops between humans, machines, and environments, driving applications from neuroplasticity recovery to precision surgery. Below is an exploration of its real-world applications, technological innovations, clinical value, and future potential.
I. Key Applications and Innovations
- Surgical Training and Precision Medical Operations
- VR Surgical Simulation:
Haptic feedback systems simulate interactions between surgical tools and tissues (e.g., vascular elasticity, tumor hardness) via force-sensing gloves and tactile skin technology, allowing surgeons to train in virtual environments. - Remote Haptic Surgery:
Robotic systems with 5G connectivity translate surgeons’ hand movements into real-time robotic actions while providing tactile feedback (e.g., heart valve tension), enabling cross-regional “haptic telepresence.”
- VR Surgical Simulation:
- Neurological and Motor Rehabilitation
- Post-Stroke Motor Recovery:
Haptic gloves (e.g., HaptX Gloves) provide real-time motion feedback via pressure arrays, combined with brain-computer interfaces (BCIs) to detect movement intent, accelerating motor cortex reorganization. - Peripheral Nerve Repair:
Skin-stretch feedback devices compensate for lost proprioception in conditions like diabetic neuropathy, reducing fall risks by alerting users to ground friction changes.
- Post-Stroke Motor Recovery:
- Multimodal Perception and Cognitive Enhancement
- Alzheimer’s Intervention:
Tactile memory activators use texture discrimination tasks (e.g., virtual sandpaper) to stimulate hippocampal and parietal cortex activity, paired with amyloid-clearing techniques to slow cognitive decline. - Autism Spectrum Disorder (ASD) Therapy:
Haptic-visual training systems link pressure-sensitive mats to VR scenarios, improving social interaction by associating tactile stimuli with emotional cues.
- Alzheimer’s Intervention:
- Telemedicine and Home-Based Rehabilitation
- Remote Diagnostics:
Portable stethoscopes with haptic sensors (e.g., Eko Core) allow remote detection of heart murmurs and lung abnormalities, matching in-person diagnostic accuracy. - Assistive Robotics:
Devices like the Tactile Cane use haptic feedback and inertial navigation to provide real-time environmental awareness for visually impaired or elderly users.
- Remote Diagnostics:
II. Technological Breakthroughs and Clinical Impact
- Haptic Encoding and Neural Mapping
- Submodal Separation:
Microelectrode arrays distinguish pressure, vibration, and temperature cues (e.g., Merkel cells for static pressure, Pacinian corpuscles for vibration), enabling biomimetic signal encoding for prosthetics. - Brain-Tactile Interfaces (BTI):
Convert haptic stimuli into thalamocortical neural oscillations (e.g., gamma-band synchronization) to manage chronic pain via targeted vibration therapy.
- Submodal Separation:
- Haptic-Driven Neuroplasticity
- Adaptive Rehabilitation Systems:
Haptic robots adjust assistance levels based on EMG signals and EEG feedback, enhancing post-stroke hand-grip precision. - Multisensory Integration:
Synchronized haptic, visual, and auditory feedback in VR activates mirror neuron systems, accelerating motor recovery.
- Adaptive Rehabilitation Systems:
- Clinical Translation of Haptic Data
- Biomarker Identification:
Tactile arrays quantify force entropy in Parkinson’s tremors, serving as objective disease progression markers. - Metabolic Correlation Models:
Machine learning links tactile sensitivity to brain glucose metabolism, predicting Alzheimer’s progression from mild cognitive impairment.
- Biomarker Identification:
III. Challenges and Future Directions
- Technical Barriers
- Personalized Haptics:
AI-driven haptic profile generators adapt feedback to individual thresholds (e.g., age-related vibration sensitivity decline). - Biocompatibility:
Biodegradable conductive hydrogels (e.g., PEDOT:PSS-Chitosan) mitigate signal decay in implantable electrodes.
- Personalized Haptics:
- Ethics and Accessibility
- Equity in Care:
Open-source platforms (e.g., OpenHaptics) and low-cost actuators democratize access to advanced haptic rehabilitation tools. - Data Privacy:
Federated learning with homomorphic encryption protects sensitive neuro-haptic data during cross-institutional collaborations.
- Equity in Care:
- Emerging Frontiers
- Quantum Haptic Sensors:
Diamond-based probes detect neuron-level strain for ultra-early Parkinson’s diagnosis. - Synthetic Tactile Organs:
3D-bioprinted tactile corpuscles restore temperature and pressure perception in burn patients. - Haptic-Metabolic Coupling:
Optogenetically engineered astrocytes release lactate in response to tactile stimuli, reversing synaptic loss post-brain injury.
- Quantum Haptic Sensors:
IV. Conclusion and Outlook
Haptic sensing is evolving from a feedback tool to a core driver of neurorehabilitation and precision medicine. Its transformative potential spans:
- Microscale Intervention: Direct modulation of ion channels and gene expression via neural interfaces.
- Multimodal Integration: Cross-brain plasticity activation through haptic-visual-auditory ecosystems.
- Global Accessibility: Democratizing advanced care via wearable haptic-cloud platforms.
As haptic encoding and biohybrid technologies advance, Haptisense may bridge digital therapeutics and neuroimmune regulation, heralding a “Haptics-as-Medicine” era.
Data sourced from public references. For collaboration or domain inquiries, contact: chuanchuan810@gmail.com