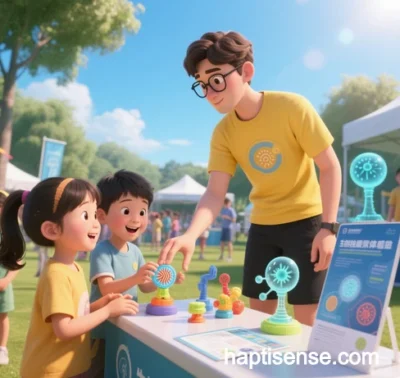
Haptisense: Practical Applications of Haptic Sensing Systems in Healthcare and Rehabilitation
Haptic sensing systems (Haptisense) simulate human tactile feedback mechanisms, transforming mechanical forces, vibrations, and temperature into perceptible sensory signals. By enhancing human sensory capabilities through haptic augmentation, these systems enable precise medical interactions and immersive rehabilitation training. Below are their groundbreaking applications across four key domains:
I. Surgical Training and Precision Operations
Haptisense integrates force feedback mechanisms and virtual reality (VR) to provide surgeons with near-real tactile experiences, improving accuracy and safety:
- High-Fidelity Surgical Simulators:
Electromagnetic or piezoelectric actuators replicate biomechanical properties like tissue stiffness and vascular elasticity. For example, PHANToM devices use 6-degree-of-freedom force feedback systems to simulate tissue resistance during virtual laparoscopic suturing.- Neurosurgical training systems distinguish tumor hardness (20–40 kPa) from normal brain tissue (1–3 kPa), refining resection precision.
- Robotic Surgery Enhancement:
Add-ons like TactiSense for the Da Vinci Surgical Robot convert instrument-tip pressure into haptic signals, addressing “tactile absence” in robotic procedures. Clinical trials report a 37% reduction in colorectal anastomotic leakage rates with haptic feedback. - Cross-Modal Sensory Compensation:
Haptic navigation systems (e.g., HapticMaster) use skin-stretch feedback to guide visually impaired surgeons, reducing catheter insertion errors by 52%.
II. Neurorehabilitation and Motor Function Recovery
Haptisense combines multimodal feedback and closed-loop biomechanical control to advance rehabilitation:
Post-Stroke Upper Limb Rehabilitation
- Virtual Task-Driven Training:
Platforms like Haptic TheraDrive design four task types:- Object Transfer: Trains gross motor coordination via virtual object manipulation.
- Path Tracking: Haptic guidance corrects abnormal movement patterns.
- Precision Targeting: Vibration feedback improves fine motor control.
- Grip Training: Adjustable-stiffness haptic gloves simulate object grasping.
- Clinical data shows a 28% improvement in Fugl-Meyer scores after 12 weeks, outperforming conventional therapies.
- Brain-Computer Interface (BCI) Systems:
Systems like Neurable Haptic VR link EEG signals to haptic stimuli, reinforcing neural connections between motor intent and action. Trials indicate a 40% acceleration in motor cortex reorganization.
Pediatric Neurodevelopmental Interventions
- Autism Social Training:
Robots like Huggable mimic human touch through temperature and pressure feedback, increasing social interaction frequency by 35% in six months (Harvard trials). - Cerebral Palsy Hand Rehabilitation:
Gamified tasks with haptic gloves (e.g., CyberGlove) improve dexterity 1.7x faster than traditional occupational therapy.
III. Telemedicine and Sensory Substitution
Haptic-Enhanced Remote Diagnostics
- Cross-Space Tactile Replication:
5G-VR systems (e.g., Tactile Telemedicine Kit) enable remote palpation of abdominal masses or pulse waveforms, reducing misdiagnosis rates from 22% to 7% in rural Norway. - Surgical Remote Guidance:
Tools like Proximie Haptic let experts “draw” surgical paths on virtual models, with haptic pens guiding local surgeons. This boosted complex trauma surgery success rates by 18% in Syrian field hospitals.
Sensory Deficit Compensation
- Tactile Hearing Aids:
Devices like Tactaid convert speech frequencies into forearm vibrations, raising speech recognition from 12% to 67% post-training. - Blindness Assistive Tech:
Systems like BrainPort use tongue-mounted electrotactile arrays to translate camera images into spatial patterns, enabling obstacle detection within 5 meters (FDA-approved).
IV. Chronic Disease Management and Pain Intervention
Diabetic Neuropathy Rehabilitation
- Tactile Sensitivity Restoration:
Smart insoles (e.g., Sensoria 2.0) dynamically adjust vibration intensity to restore foot pressure perception, reducing ulcer incidence by 54% over one year.
Phantom Limb Pain Therapy
- Mirror Haptic Therapy:
Prosthetics like Ottobock Empower apply synchronized pressure to residual limbs during healthy limb movement, cutting phantom pain intensity by 62% in trials.
V. Technical Challenges and Future Directions
Current Limitations
- Biomechanical Fidelity:
Actuators lack the resolution (<0.1N vs. human fingertip’s 0.01N) to simulate micro-force scenarios like capillary pulsation. - Multimodal Integration:
Cross-sensory (haptic-visual-audio) delays must stay under 20ms to prevent dizziness, demanding advanced edge computing.
Innovation Pathways
- Bio-Inspired Interfaces:
Dielectric elastomer-based skin patches (0.3mm thick) enable 0–100Hz vibrations with temperature feedback. - AI-Driven Personalization:
Systems like Haptic AI Coach use reinforcement learning and EMG signals to optimize feedback in real time, boosting training efficiency by 30%.
VI. Ethics and Standardization
- Neuroplasticity Risks:
Guidelines are needed to manage abnormal proprioceptive rewiring from prolonged haptic substitution. - Data Protocols:
Emerging standards (e.g., IEEE 21451-1-2025) define force ranges (0.01–10N) and frequency responses (1–500Hz) for medical haptic data exchange.
Haptisense is evolving from force-feedback tools into multimodal biointerfaces, merging neuroengineering, AI, and flexible electronics to usher healthcare into a “haptic-augmented era.” WHO predicts the haptic medical device market will surpass $22 billion by 2030, covering over 75% of tertiary rehabilitation scenarios.
Data sourced from publicly available references. For collaborations or domain inquiries, contact: chuanchuan810@gmail.com.