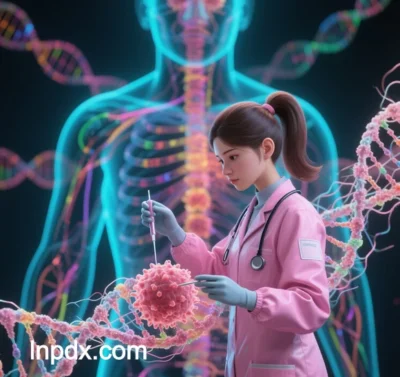
LNP-Dx: Applications in Genetic Testing and Early Cancer Diagnosis
LNP-Dx (Lipid Nanoparticle Diagnostics), an advanced derivative of nucleic acid delivery systems, leverages the targeting, protective, and signal-amplifying capabilities of lipid nanoparticles (LNPs) to redefine genetic testing and early cancer diagnostics. Below is an analysis of its technical principles, applications, clinical translation, and challenges:
I. Technical Principles and Design Advantages
- Nucleic Acid Probe Delivery
- CRISPR-Cas Integration: LNPs deliver Cas12a with crRNA to detect tumor-associated mutations (e.g., KRAS G12D). Target recognition triggers trans-cleavage activity, releasing fluorescent or electrochemical signals (sensitivity: fg/mL level).
- Multiplex Detection: STAgR systems enable multi-target gRNA libraries, allowing single LNPs to detect >10 tumor biomarkers (e.g., EGFR, TP53 mutations in ctDNA).
- Targeted Delivery and Signal Enhancement
- Active Targeting: LNPs conjugated with tumor-specific ligands (e.g., anti-EpCAM antibodies, folate receptors) enrich low-abundance targets like circulating tumor cells (CTCs) or exosomes.
- EPR Effect: Enhanced permeability and retention in tumor vasculature amplify localized signals, improving signal-to-noise ratios.
- Stability and Multiplexing
LNPs protect nucleic acid probes from nuclease degradation, extending reagent shelf life. Encapsulating differently labeled probes enables parallel detection of multiple targets.
II. Core Applications in Early Cancer Diagnosis
- Liquid Biopsy Advancements
- Ultra-Sensitive ctDNA Detection: CRISPR-Cas13a with RPA isothermal amplification detects low-frequency mutations (e.g., EGFR T790M at 0.1%) in blood, surpassing traditional ddPCR sensitivity.
- Exosome Analysis: CD63 aptamer-modified LNPs capture tumor exosomes, releasing miRNA-21 or lncRNA HOTAIR for real-time nanopore sequencing.
- In Situ Molecular Imaging
- Tumor Microenvironment Probes: pH-sensitive LNPs release near-infrared probes in acidic tumors, enabling live imaging of liver metastases via APOE adsorption.
- Epigenetic Biomarkers: dCas9-EZH2 fusion proteins target methylation markers (e.g., SEPT9) for early colorectal cancer diagnosis via chromatin conformation analysis.
- Multi-Omics Integration
- Genome-Epigenome Profiling: Simultaneously detect mutations (e.g., BRCA1), methylation (e.g., MGMT), and copy number variations in a single sample.
- Drug Resistance Monitoring: siRNA libraries delivered via LNPs screen resistance pathways (e.g., PI3K/AKT), combined with single-cell sequencing to resolve clonal heterogeneity.
III. Clinical Translation and Case Studies
- Early Screening Tools
- Lung Cancer Methylation Kit: LNP-encapsulated SERPINB5 probes detect early biomarkers in sputum via electrochemical sensors (specificity >95%).
- Breast Cancer CTC Profiling: EpCAM-targeted LNPs capture CTCs, releasing PD-L1 mRNA and HER2 DNA via microfluidics to guide immunotherapy.
- Companion Diagnostics
- PARP Inhibitor Sensitivity: CRISPRi libraries silence homologous recombination genes (e.g., BRCA2) in organoids to evaluate olaparib efficacy.
- Immunotherapy Biomarkers: Fluorescent RNA aptamers quantify IFN-γ mRNA in tumor-infiltrating lymphocytes (TILs), predicting PD-1 inhibitor response.
- Emerging Technologies
- AI-Driven Monitoring: Graph neural networks (GNNs) analyze multi-omics data to predict pancreatic cancer progression with 30% higher accuracy.
- Organ-on-a-Chip Integration: Liver chips with LNP-Dx simulate metastatic niches to assess CTC colonization and recurrence risks.
IV. Challenges and Innovations
- Specificity Challenges
- Off-Target Signals: Cell-free DNA and non-tumor exosomes cause false positives; solutions include high-fidelity Cas variants (e.g., HypaCas13) or dual-validation probes.
- Matrix Interference: Mucins and nucleic acid-binding proteins in sputum/blood reduce probe efficiency; optimize LNP surface PEG density and hydrophobicity.
- Standardization and Accessibility
- Lyophilization Stability: Current LNP-Dx reagents require 4°C storage; develop room-temperature formulations (e.g., trehalose vitrification).
- Cost-Effective Production: Replace expensive microfluidics with paper-based chips or 3D printing for resource-limited regions.
- Ethical and Regulatory Frameworks
- Data Privacy: Blockchain-based anonymization secures multi-omics genetic data.
- Validation Standards: WHO is advancing ASSURED-compliant guidelines for LNP-Dx sensitivity/specificity thresholds.
V. Future Directions
- Closed-Loop Diagnostic-Therapeutic Systems
Integrate CRISPR-Cas12 detection with siRNA release (e.g., KRAS mutation suppression) for simultaneous diagnosis and treatment. - Synthetic Biology Integration
Design self-activating LNPs that release STING agonists upon detecting TP53 mutations, combining diagnostics with immune activation. - Multi-Scale Spatial Diagnostics
Use barcoded probes delivered by LNPs to map pre-cancerous lesions via spatial transcriptomics, reconstructing tumor evolution in 4D.
Conclusion
LNP-Dx is revolutionizing early cancer diagnostics by merging nucleic acid delivery, signal amplification, and multi-omics analysis. Advances in CRISPR, AI modeling, and low-cost manufacturing could make it a universal screening tool within 5–10 years. However, standardization, ethical governance, and accessibility remain critical hurdles, demanding interdisciplinary collaboration and policy innovation.
Data sourced from public references. For collaboration or domain inquiries, contact: chuanchuan810@gmail.com