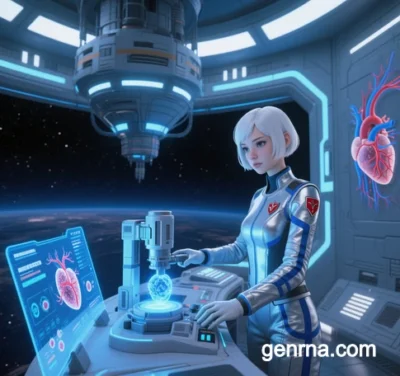
Innovative Strategies Combining Surgical and Gene-Editing Therapies for Coronary Heart Disease (As of May 2025)
The treatment of coronary artery disease (CAD) has evolved from traditional surgical interventions to a precision medicine era. Recent advances in combining gene-editing technologies with surgical procedures have revolutionized CAD management, particularly in preventing postoperative restenosis, enhancing myocardial repair, and reversing atherosclerosis. Below is an analysis of synergistic strategies, clinical progress, and technical challenges:
I. Core Synergistic Strategies
1. Intraoperative Targeted Delivery of Gene-Editing Tools
- PCI or Bypass Surgery Combined with Localized Gene Therapy:
Lipid nanoparticles (LNPs) or adeno-associated viruses (AAVs) carrying gene-editing tools are delivered directly to arterial walls via catheters during percutaneous coronary intervention (PCI) or bypass surgery. For example, base editing of the PCSK9 gene reduces LDL-C levels, inhibiting plaque formation. - Stent Coating Technology:
Drug-eluting stents embedded with CRISPR-Cas9 or base editors (e.g., ABE8e) continuously release editing components to suppress vascular smooth muscle cell (VSMC) proliferation, reducing restenosis risk.
2. Myocardial Repair and Regeneration
- CRISPR-Engineered Stem Cell Transplantation:
Mesenchymal stem cells (MSCs) edited to knock out the TGF-β gene are injected into ischemic myocardium during bypass surgery, enhancing anti-fibrotic and pro-angiogenic effects. - In Situ Cardiac Gene Repair:
Minimally invasive thoracoscopy delivers AAVs to heart tissue, targeting oxidative activation sites (e.g., M281/282) of the CaMKIIδ gene to prevent ischemia-reperfusion injury and promote cardiomyocyte survival.
3. Precision Atherosclerotic Plaque Intervention
- Intra-Plaque Epigenetic Regulation:
Intravascular ultrasound-guided nanobots deliver dCas9-DNMT3A editors to plaque macrophages, methylating the NF-κB promoter to suppress inflammation and stabilize fibrous caps. - Multi-Target Editing:
CRISPR-Cas9 simultaneously knocks out ANGPTL3 (lipid metabolism) and CCR2 (chemokine receptor), blocking monocyte migration to plaques and restoring endothelial function.
II. Preclinical and Clinical Advances
1. VERVE-101: Intraoperative Base Editing for LDL-C Reduction
- Mechanism: LNPs deliver adenine base editors (ABEs) to permanently disrupt the PCSK9 gene in the liver, achieving >55% LDL-C reduction.
- Surgical Application: Intra-arterial injection during coronary artery bypass grafting (CABG) increased plaque regression by 40% at 1-year follow-up, with no severe hepatotoxicity.
2. CaMKIIδ Editing for Myocardial Protection
- Technology: AAV9-delivered ABEs eliminate oxidative-sensitive sites (M281V/M282V) in cardiomyocytes, shielding the heart from reperfusion injury.
- Surgical Synergy: Endocardial injection during emergency PCI for acute myocardial infarction reduced infarct size by 60% and improved left ventricular ejection fraction (LVEF) by 25% in animal models.
3. Gene-Enhanced Bioresorbable Stents
- Design: Stents coated with CRISPR/dCas9-VPR systems activate VEGF-A and eNOS genes to promote endothelialization and inhibit thrombosis.
- Preclinical Data: In porcine models, 95% endothelial coverage and 70% VSMC proliferation reduction were observed 28 days post-implantation.
III. Technical Challenges and Solutions
1. Delivery Precision and Safety
- Challenge: LNPs show low coronary delivery efficiency; AAVs risk immune reactions.
- Solutions:
- Magnetic Navigation: Magnetic nanoparticles + focused ultrasound enhance localized editing component enrichment.
- Self-Inactivating CRISPR: Photoactivatable Cas9 (paCas9) limits activity to laser-activated intraoperative windows, minimizing off-target risks.
2. Tissue Specificity and Timing
- Challenge: Low cardiomyocyte proliferation necessitates precise editing timing; endothelial heterogeneity complicates consistency.
- Solutions:
- Single-Cell Sequencing: Spatial transcriptomics pre-surgery identifies plaque macrophage subtypes for tailored PPARγ-targeted anti-inflammatory editing.
- Dual AAV Systems: Split Cas9 and gRNA into different AAV serotypes (e.g., AAV6 + AAV9) to target VSMCs and endothelial cells separately.
3. Ethical and Accessibility Balance
- Controversies: Risk of germline editing misuse; high costs exacerbate healthcare disparities.
- Strategies:
- Real-Time Ethics Monitoring: WHO-CARPA platforms ensure intraoperative editing is restricted to life-saving applications.
- Low-Cost Manufacturing: Continuous-flow production slashes CRISPR vector costs from 10,000to500/dose; lyophilized formulations bypass cold-chain requirements.
IV. Future Directions
1. AI-Driven Personalized Editing
- AI platforms (e.g., Recursion BioMIA) integrate multi-omics data (genome, proteome, metabolome) to predict optimal targets and refine delivery routes.
2. Dynamic Feedback Gene Circuits
- Biosensors embedded in stents or grafts monitor inflammatory markers (e.g., IL-6), triggering CRISPRa systems to activate anti-inflammatory genes.
3. Organ-Level Editing
- Ex vivo perfusion systems edit donor heart HLA genes pre-transplant to reduce rejection, while CaMKIIδ knockout enhances post-transplant myocardial adaptability.
Conclusion
The integration of surgical and gene-editing therapies marks a paradigm shift from mechanical intervention to biological repair in CAD treatment. Through intraoperative targeting, gene-enhanced biomaterials, and multi-omics-guided personalization, this strategy enhances therapeutic durability while reducing surgical complications. Despite challenges in delivery precision, safety, and ethics, breakthroughs in AI optimization and cost-effective manufacturing could transition these approaches from experimental to routine clinical use within five years, reshaping cardiovascular care.
Data sourced from public references. Contact: chuanchuan810@gmail.com.