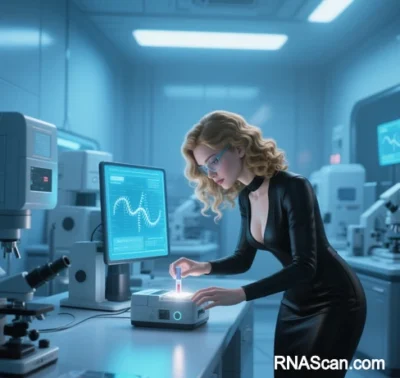
RNA-Scan: Spatial Transcriptomics and Spatiotemporal RNA Dynamics Analysis
RNA localization and dynamics are central to cellular function regulation. Recent advances in spatial transcriptomics (ST) enable researchers to analyze RNA distribution and dynamics at subcellular to tissue scales. RNA-Scan, an integrated experimental strategy combining high-resolution localization, dynamic tracking, and multi-omics analysis, has emerged as a critical tool for decoding RNA spatiotemporal biology. Below, we explore its technical principles, applications, and future directions.
I. Core Components of RNA-Scan Technology
- Subcellular RNA Localization Technologies
- APEX-Seq: Developed by Stanford researchers, this live-cell RNA tagging method uses genetically encoded APEX2 peroxidase to biotinylate RNA in specific subcellular regions (e.g., mitochondrial matrix, ER membrane). Paired with sequencing, it maps RNA localization at near-nanometer resolution, capturing transient changes like nucleolar RNA relocalization during oxidative stress.
- Salus-STS: A next-generation sequencing system optimized for probe capture efficiency, achieving micron-level resolution to differentiate RNA subcompartments (e.g., neuronal dendrites vs. axons).
- Dynamic RNA Tracking
- VISTA Algorithm: Integrates RNA velocity and spatial proximity data to predict directional gene expression patterns. For example, in tumor microenvironments, VISTA identifies invasion-promoting signals (e.g., VEGF) and traces their cellular origins.
- Metabolic Labeling: Uses 4-thiouridine (4SU) pulse-chase labeling to track RNA synthesis/degradation dynamics, revealing mechanisms like rapid nuclear export of chemokine mRNAs during inflammation.
- Multi-Omics Integration
- Patho-DBiT: A spatial whole-transcriptome platform compatible with clinical FFPE samples, detecting mRNA, lncRNA, splice variants, and SNVs. Its single-cell version maps tumor clonal evolution (e.g., TP53 mutant subclones in lymphoma).
- Stereo-seq: Combines CID (chemical interruption) labeling and PolyT probes for single-molecule RNA capture on tissue sections. Its latest iteration enables protein-RNA co-localization analysis in translation hotspots (e.g., neuronal synapses).
II. Applications of Spatiotemporal RNA Analysis
- Developmental Biology: Spatiotemporal Logic of Cell Fate Determination
- In mouse limb development, Salus-STS reveals that Sonic hedgehog (Shh) mRNA gradients directly regulate ZPA (zone of polarizing activity) formation, with RNA accumulation preceding protein signal diffusion.
- In Drosophila oocytes, VISTA reconstructs Bicoid mRNA transport waves constrained by microtubule networks.
- Neuroscience: Synaptic Plasticity and RNA Metabolism
- APEX-Seq shows Arc/Arg3.1 mRNA clustering at activated synapses within 30 minutes of LTP induction, regulating AMPA receptor insertion via local translation.
- Metabolic labeling in Alzheimer’s models links prolonged axonal retention of Tau-related mRNAs to early neurofibrillary tangle formation.
- Cancer Biology: Tumor Microenvironment Heterogeneity
- Integrative scRNA-seq/ST analysis in lung cancer identifies EMT transition cells at invasion fronts expressing fibronectin mRNA, recruiting pro-fibrotic macrophages via autocrine WNT signaling. Patho-DBiT localizes these mRNAs to pseudopod contact zones.
- Stereo-seq in breast cancer liver metastases captures RNA exchange between CTCs and endothelial cells, including exosomal miR-21 transfer.
- Infection Immunology: Viral RNA Manipulation
- In SARS-CoV-2-infected cells, VISTA maps viral RNA (e.g., N-protein coding regions) to ER-mitochondria contact sites (MAMs), coinciding with localized suppression of MAVS mRNA.
- Single-cell ST analysis of HIV reservoirs reveals proviral DNA integration near host SATB1 transcription sites, promoting viral reactivation.
III. Advantages and Challenges
Aspect | Advantages | Challenges |
---|---|---|
Resolution | Subcellular to single-molecule precision | Limited temporal resolution in live imaging |
Multi-Omics | Simultaneous RNA/protein/SNV detection | Data standardization and algorithm integration |
Clinical Utility | Compatibility with FFPE samples | High costs hinder large-scale studies |
Dynamic Analysis | Quantifies RNA synthesis/degradation rates | Phototoxicity limits long-term live-cell imaging |
IV. Future Directions
- Ultra-Resolution Live Imaging
Develop CRISPR-Cas13-based RNA tracking systems with photoactivatable probes (e.g., PATRONUS) for second-scale temporal and 100 nm spatial resolution. - Computational Innovations
- Build differential equation models of RNA spatiotemporal dynamics to predict morphogen gradient self-organization.
- Train multimodal deep learning frameworks (e.g., SpaGNN) to infer cell-cell signaling from ST data.
- Clinical Translation
- Establish spatial RNA biomarker panels (e.g., Patho-DBiT-guided lymphoma SNV maps) for targeted therapies.
- Integrate liquid biopsy with ST to monitor metastasis via CTC analysis.
- Synthetic Biology Synergy
Engineer artificial RNA localization signals (e.g., axon-targeting motifs) for precise mRNA delivery in gene therapy.
Conclusion
RNA-Scan’s “localization-dynamics-integration” framework is redefining RNA spatiotemporal biology by:
- Uncovering RNA’s role as a spatial information carrier (e.g., local signaling hubs).
- Decoding molecular dialogues in disease microenvironments (e.g., tumor-immune crosstalk).
- Advancing precision medicine from “cell atlases” to “spatial atlases.”
With advancements in quantum dot labeling, single-molecule sequencing, and AI-driven imaging, real-time RNA dynamics in living tissues may soon become a reality, offering unprecedented insights for biomedicine.
Data sourced from public references. For collaboration or domain inquiries, contact: chuanchuan810@gmail.com