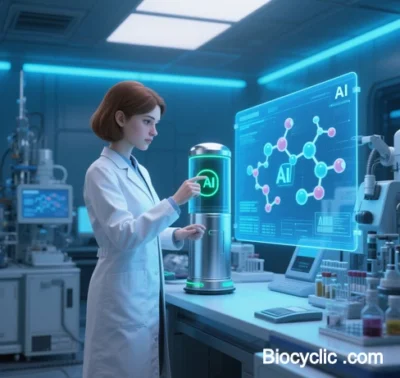
Bio-Cyclic: Building a Symbiotic Circular Economy Model
A Systemic Economic Paradigm
Bio-Cyclic is an economic framework designed to mimic natural material cycles and energy flows, transforming human production and consumption systems into closed-loop, self-regenerating ecosystems. By replacing the linear “extract-produce-waste” model with a dynamic “resource-product-renewable resource” balance, it aligns economic activity with Earth’s biogeochemical rhythms. This framework is explored through four dimensions: theoretical foundations, implementation pathways, technological enablers, and future challenges.
I. Theoretical Foundations: Translating Nature’s Laws to Industry
1. Redesigning Circular Logic
- Metabolic Homology Principle: Industrial processes must mirror biological metabolism, ensuring outputs return to the biosphere (e.g., compost) or circulate infinitely in the technosphere (e.g., metal recycling).
- Energy Hierarchy Alignment: Optimize industrial energy use through distributed renewables and waste-heat recovery, inspired by ecological energy transfer efficiency (~10%).
2. Innovation in Design
- Cradle-to-Cradle®: Products are designed for disassembly, using biodegradable “biological nutrients” or infinitely recyclable “technical nutrients.” Example: Interface’s modular carpet recycling.
- Biomimicry: Engineering solutions inspired by 380 million years of evolution, such as termite mound-inspired ventilation systems and lotus leaf-inspired self-cleaning materials.
II. Implementation Pathways: Closed-Loop Networks from Soil to Space
1. Agriculture & Food Systems
- Closed-Loop Agro-Industrial Complexes:
- Ajinomoto’s “Bio-Cycle” converts CO₂ to fertilizer, processes bagasse into amino acids, and uses wastewater for biogas power, achieving zero waste.
- Philippine rice farming integrates biochar production, insect protein feed, and manure recycling, boosting nitrogen efficiency from 30% to 85%.
- Food Waste Regeneration: Anaerobic digestion converts organic waste into biogas (energy) and digestate (fertilizer), exemplified by Copenhagen’s Amager Resource Centre powering 100,000 homes annually.
2. Materials & Manufacturing Revolution
Material Category | Innovation | Environmental Impact |
---|---|---|
Bio-Based Plastics | Braskem’s sugarcane-derived polyethylene reduces carbon footprint by 70% vs. petroleum-based plastics. | Degrades in 3–5 years vs. 450 years for conventional plastics. |
Mycelium Composites | Ecovative grows packaging and construction materials from agricultural waste, decomposing naturally in 28 days. | Reduces reliance on polystyrene and fossil fuels. |
Biomineralized Materials | BioMason’s microbially grown bricks cut energy use by 90% with zero CO₂ emissions. | Sustainable alternative to traditional masonry. |
3. Urban Metabolism Optimization
- Water-Energy-Waste Integration: Singapore’s NEWater project reclaims 40% of wastewater via membrane bioreactors, while incinerator ash is repurposed for land reclamation (95% material recovery).
- Ecosystem-Integrated Architecture: Milan’s Bosco Verticale skyscraper absorbs 19 tons of CO₂ annually and boosts biodiversity through green walls and rainwater harvesting.
III. Technological Enablers
1. Biotech Innovation
- Synthetic Biology: CRISPR-engineered microbes degrade plastics (PETase enzymes) and absorb heavy metals (biofilm engineering).
- Digital Twin Modeling: The EU’s CircularTwins platform optimizes waste management in real time, increasing recycling efficiency by 35%.
2. Blockchain for Circularity
- Circularise: Tracks plastic lifecycle transparency across 200+ companies, including Philips and Volkswagen.
- ReSource: Converts biochar carbon sequestration into tradable credits, incentivizing regenerative farming.
IV. Challenges & Future Directions
1. Current Barriers
- Cost Disparities: Bio-based materials remain pricier than fossil-based alternatives (e.g., PLA costs double PP). Carbon taxes and green procurement policies are critical.
- Regulatory Fragmentation: Divergent biodegradability standards (e.g., EN13432 vs. ASTM D6400) hinder global trade.
- Infrastructure Lock-In: Legacy linear systems (landfills, incinerators) slow transition; phased retirement strategies are needed.
2. Frontier Innovations
- Space Biocycling: NASA’s CELSS uses algae photobioreactors to regenerate oxygen and food for Mars missions.
- Quantum-Bio Interfaces: Quantum dots enhance algal photosynthesis, tripling carbon fixation rates while producing biofuels.
3. Policy Levers
- Extended Producer Responsibility (EPR 3.0): Mandates full lifecycle ecological accountability, e.g., France’s 30% recycled plastic requirement in electronics.
- Biodiversity Finance: Natural capital bonds monetize ecosystem services like mangrove carbon sinks to fund circular projects.
Conclusion
Bio-Cyclic represents not just a technological shift but a civilizational evolution—from “conquerors of nature” to “ecological collaborators.” By harmonizing industrial and biological metabolisms, embedding regeneration into product design, and transforming cities into circular nodes, humanity can achieve lasting symbiosis with Earth’s living systems.
Data sourced from publicly available references. For collaborations or domain inquiries, contact: chuanchuan810@gmail.com.