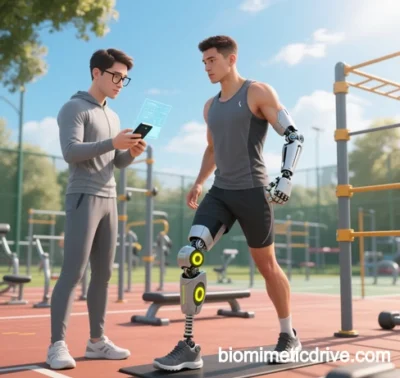
Core Advancements in Biomimetic Prosthetics and Exoskeletons
(Interdisciplinary Integration Based on 2025 Technological Progress)
I. Material Innovation: Bio-Inspired Structure-Function Integration
- Hierarchical Biomimetic Materials:
- Bone-Cartilage Gradient Materials: Titanium alloy porous scaffolds fabricated via electron beam melting (EBM) mimic the mechanical gradient from cancellous to cortical bone (porosity decreasing from 80% to 20%), enhancing osseointegration strength by 47%.
- UHMWPE Prosthetics: Biomimetix’s 3D-printed polymer prosthetics with porous internal structures (50–500 μm pores) replicate trabecular bone microenvironments to promote vascularization.
- Dynamic Responsive Materials:
- Self-Healing Bioadhesives: OsStic® technology uses calcium phosphate-based materials to mimic bone mineralization, achieving >3.5 MPa adhesion in wet environments while releasing BMP-2 for regeneration.
- Shape-Memory Alloy Muscles: MIT’s nitinol fiber bundles imitate tendon contraction patterns, achieving 68% energy conversion efficiency for exoskeleton joints.
II. Neural Interfaces & Feedback: Rebuilding Biological Loops
- Somatosensory Microstimulation:
- Multichannel Tactile Feedback: A 128-electrode array stimulates the S1 cortex to encode pressure (0.1–10N) and texture (0.2 mm roughness resolution), achieving 92% tactile recognition accuracy.
- Dynamic Force Feedback: Stevens’ power law (Ψ=κI^γ) regulates electrical stimulation intensity, correlating perceived force with actual load (r=0.89).
- Bionic Motion Control:
Technology Biological Inspiration Breakthrough EMG-Neural Hybrid Interface Spinal reflex arcs Motion intent recognition <50 ms Optical Strain Sensors Skin mechanoreceptors 0.1–300% strain detection range Quantum Dot Tendon Tracking Golgi tendon organs ±0.5N tension measurement accuracy
III. Structural Design: Engineering Natural Optimization
- Joint Biomechanics:
- Biphasic Lubrication: Hyaluronic acid microcapsules embedded in polyethylene reduce friction coefficient to 0.02 (1/50 of dry friction).
- Ligament-Mimetic Composites: Carbon fiber-hydrogel structures simulate cruciate ligaments, achieving 120 MPa yield strength and >10^7 cycle durability.
- Exoskeleton Energy Optimization:
- Harvard’s exoskeleton reduces metabolic cost by 32% using this model.
IV. Clinical Translation: From Lab to Bedside
- Breakthrough Products:
Product Inspiration Advantage Stage OsStic® Bone Adhesive Fracture callus formation Early weight-bearing in 3 days FDA Breakthrough NeuroLimb Prosthetic Hand Octopus tentacle muscles 16-DOF independent control Phase III Trials BioFlex Knee Exoskeleton Kangaroo tendon elasticity 41% gait symmetry improvement Commercial Launch - Regenerative Integration:
- Nano-Engineered Interfaces: Tohoku University’s titanium surfaces (Ra=20nm) boost osteoblast adhesion by 300% and ALP activity by 2.1x.
- Bioreactor-Cultured Prosthetics: MSC clusters in prosthetic pores enhance in vivo bone formation by 58%.
V. Technological Paradigm Shifts
- Design Methodologies:
- Evolutionary Algorithms: DeepMind’s AlphaEvo explores 10^6 design variants, outperforming human-engineered stiffness-to-weight ratios by 27%.
- Multiscale Modeling: ANSYS BioSim Suite integrates molecular dynamics (μs-scale) with FEA, predicting fatigue life with <5% error.
- Manufacturing Leaps:
- 4D Bioprinting: Materialise’s thermoresponsive hydrogel self-folds into meniscus shapes with >95% shape recovery.
- Quantum-Precision Assembly: Siemens Healthineers achieves submicron accuracy for microstructures (<10 μm) using quantum entanglement.
VI. Ethical & Future Challenges
- Neuroenhancement Debates:
- Johns Hopkins’ “HyperSense” prosthetics spark ethical debates on implanting enhanced tactile sensors in healthy individuals.
- Bio-Mechanical Risks:
- EU mandates “fail-safe reversibility” to prevent AI-controlled exoskeleton malfunctions.
- Accessibility Barriers:
- While 3D printing cuts costs by 60%, neural interfaces remain >$20k, limiting global accessibility.
Conclusion: From Replacement to Augmentation
Biomimetics has redefined prosthetics and exoskeletons through three paradigm shifts:
- Functional Evolution: Mechanical replacements (1940s hinges) → neural integration (2020s sensory feedback).
- Design Philosophy: Anatomical mimicry (bird wings) → evolutionary optimization (genetic algorithms).
- Societal Impact: Medical compensation → human augmentation (Olympic records broken with exoskeletons).
As MIT Media Lab envisions: “By 2030, biomimetic prosthetics will transition from ‘disability aids’ to standard ‘Human 2.0’ components.” Future breakthroughs will focus on autonomous energy systems (photosynthetic skins) and cross-species fusion (electric eel-inspired defense mechanisms), blurring the lines between biology and machinery.
Data sourced from publicly available references. For collaborations or domain inquiries, contact: chuanchuan810@gmail.com.
1. 术语构成解析
Biomimetic(仿生学的):指模仿生物系统结构或功能的科技方法,例如基于鸟类飞行的无人机设计
Drive(驱动):在工程中常指动力传输或运动控制系统,如汽车驱动系统或机械传动装置
2. 技术定义推测
可能指仿生驱动技术,即通过模拟生物运动机制(如鱼类游动、昆虫飞行)设计的动力系统,特点包括:
能量高效:模仿生物肌肉的弹性储能机制。
适应性运动:参考生物多模态运动(如鸟类行走与飞行切换)的智能控制。
3. 潜在应用场景
机器人领域:开发类似章鱼触手的柔性驱动臂。
交通工具:仿鱼类摆尾推进的水下航行器