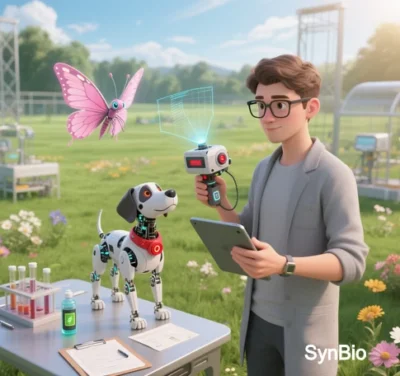
The Pivotal Role of High-Precision Sensors in Synthetic Biology
High-precision sensors are central to achieving dynamic control, real-time monitoring, and precision design in synthetic biology, with applications spanning fundamental research to industrial deployment. Below, we dissect their multidimensional value across five domains, supported by key technological breakthroughs and case studies (2020–2025).
I. Real-Time Navigation for Metabolic Engineering
High-precision sensors enable dynamic feedback to optimize cell factories by capturing metabolic flux changes:
- Bottleneck Identification:
Fluorescent RNA sensors (e.g., HyPerion) or FRET-based protein conformation sensors track rate-limiting enzyme activity (e.g., P450 oxidases).
Case Study: NADPH/ATP dual-channel sensors identified mevalonate kinase as a bottleneck in artemisinin pathways, boosting yield 3.2-fold. - Resource Competition Quantification:
Orthogonal sensors (e.g., ribosome binding site variants) monitor host vs. heterologous resource use (ATP/NADH), preventing metabolic imbalance.
Case Study: In E. coli taxol precursor pathways, orthogonal sensors revealed host TCA cycle ATP consumption >40%, enabling dynamic gene downregulation for yield optimization.
II. Precision Triggers for Genetic Circuit Control
Sensors empower environment-responsive, logic-driven circuits:
- Molecular Logic Gates:
RNA toehold switches or CRISPR-dCas9 sensors process multi-input signals (pH, temperature, metabolites) to execute AND/OR/NOT operations.
Case Study: MIT’s dual EGFR/HER2 sensor system triggers apoptosis in dual-positive cancers with >99% specificity. - Dynamic Oscillation:
Optogenetic (e.g., EL222) or metabolite-responsive (e.g., LuxR/AHL) sensors enable pulsatile gene expression.
Case Study: Repressilator circuits with arginine sensors mimic β-cell insulin pulses, reducing glucose fluctuations to <5%.
III. Revolutionary Tools for Environmental & Medical Monitoring
Sensors bridge lab research to real-world applications:
- Pollutant Detection:
Whole-cell biosensors (e.g., engineered Pseudomonas putida) detect ppb-level aromatic hydrocarbons while degrading them.
Case Study: Protein sensor strips detect benzene derivatives in wastewater at 0.1 nM sensitivity, 10x faster than HPLC. - Medical Diagnostics:
Nanobody or RNA aptamer sensors track biomarkers (e.g., tumor microenvironment H₂O₂) in vivo.
Case Study: HyPerion-enabled transgenic mice image cancer stem cells at single-cell resolution.
IV. Guardians of Synthetic System Stability
Sensors ensure long-term reliability through evolution monitoring and adaptive regulation:
- Mutation Surveillance:
CRISPR-Cas9 sensors detect plasmid loss or critical mutations (e.g., antibiotic resistance genes), activating toxin-antitoxin circuits.
Case Study: Sensor-equipped E. coli maintained 95% plasmid retention over 100 generations vs. 60% in controls. - Environmental Resilience:
Dual temperature/salinity sensors dynamically regulate compatible solute synthesis (e.g., ectoine).
Case Study: Bacillus subtilis engineered with ectABC sensors sustained 85% fermentation efficiency under 15% NaCl.
V. Cornerstones of Standardized Platforms
Sensors drive synthetic biology toward predictable engineering:
- Modular Interfaces:
SEVA 3.0 standardized sensor modules (e.g., BioBrick promoter-RBS) enable plug-and-play circuit assembly.
Case Study: pH sensor integration reduced lactate production variability across labs from ±50% to ±5%. - Digital Twins:
AI models (e.g., DeepKINETICS) generate dynamic cell factory mirrors using single-cell sensor data (metabolites, gene expression noise).
Case Study: Yeast digital twins increased butanol pathway success rates from 20% to 65% in first-round designs.
Challenges & Future Directions
Challenge | Solution | Technology/Case |
---|---|---|
Host background noise | Orthogonal sensors (XNA-dependent) | Cell-free transcription-translation |
Limited dynamic range | Cascaded signal amplification | Enzyme-linked aptasensors (E-aptasensors) |
Cross-species compatibility | Machine learning-guided universal sensors | AlphaFold2-predicted aptamer binding |
Future integration with synthetic-natural system theory and non-equilibrium thermodynamics will propel synthetic biology from “programmable” to “evolvable” systems.
Data sourced from public references. Contact: chuanchuan810@gmail.com.