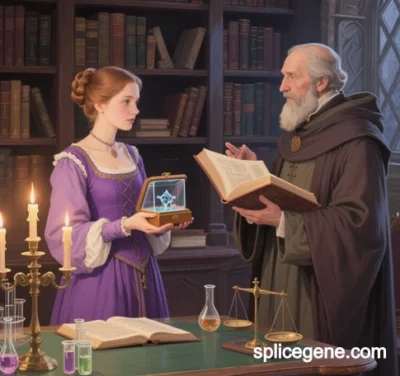
Splice Genes in Protein Diversity and Tissue-Specific Regulation: Advances and Applications
I. Biological Basis of Alternative Splicing and Protein Diversity
1. Prevalence and Molecular Mechanisms
Alternative splicing, a central post-transcriptional regulatory mechanism, generates multiple mRNA isoforms from a single gene by selectively combining exons and introns, ultimately translating into functionally diverse proteins.
- Dominance in the Human Genome: Approximately 95% of multi-exon genes undergo alternative splicing, far exceeding lower eukaryotes like yeast (only 6% of genes contain introns), highlighting its role in biological complexity.
- Splicing Signals and Regulatory Elements:
- Core Splicing Signals: Include the 5′ splice site (5’SS), 3′ splice site (3’SS), and branch point.
- Cis-Regulatory Elements**: Exonic/intronic splicing enhancers (ESEs/ISEs) and silencers (ESSs/ISSs) dynamically regulate splicing by binding splicing factors (e.g., SR proteins, hnRNPs).
- Quantifying Protein Diversity: A single gene can produce dozens of splice variants. For example, the human DSCAM gene generates over 38,000 isoforms, vastly surpassing the 48 variants in its fruit fly homolog.
2. Spatiotemporal Dynamics of Splicing
- Developmental Stage Specificity: Splicing factors (e.g., Nova, FOX family) drive isoform switching in critical pathways (e.g., Wnt, Notch) during embryogenesis.
- Cell-Type Specificity: Neurons exhibit high splicing activity (e.g., Neurexin gene regulation of synaptic plasticity), while immune cells (e.g., T cells) modulate activation states via CD45 isoform switching.
II. Molecular Mechanisms of Tissue-Specific Splicing
1. Epigenetic and Chromatin Regulation
- Histone Modifications: H3K36me3 marks active transcription regions, recruiting splicing factors (e.g., PTB) to promote exon skipping. H3K4me3 extends spliceosome assembly by pausing RNA polymerase II.
- Chromatin Accessibility: Open chromatin regions (e.g., near promoters) facilitate splicing factor binding, while heterochromatin suppresses splice variant production.
2. Tissue-Specific Splicing Factor Networks
- Muscle: MBNL1/2 binds UG-rich sequences to regulate TTN (titin) splicing, impacting muscle fiber elasticity. Dysregulation causes myotonic dystrophy.
- Brain: NOVA proteins target neuron-specific exons (e.g., GABA receptor genes), shaping neurotransmitter receptor diversity.
- Liver: HNRNPA1/A2B1 suppresses APOB exon 26 retention, generating the shorter APOB48 isoform for lipid metabolism.
III. Cutting-Edge Technologies for Splicing Analysis
1. Deep Learning-Driven Splicing Prediction
- SpliceAI: A 32-layer neural network predicts splice sites and variant impacts (Δ score), identifying pathogenic splicing in Alzheimer’s disease (e.g., PLCg1 exon 26).
- SpliceTransformer: Combines Transformer architecture with multi-modal data (sequence, epigenetics, tissue expression) for tissue-specific predictions (20% accuracy gain over traditional models).
- DeepCode: Integrates RNA secondary structures and splicing factor binding sites to predict cancer-associated splicing (e.g., BRCA1 exon 18 skipping).
2. High-Throughput Splicing Profiling
- Long-Read Sequencing (PacBio/Nanopore): Resolves full-length transcript isoforms, revealing complex splicing patterns (e.g., circular RNAs, chimeric transcripts).
- Single-Cell Splicing Atlas: 10x Genomics and Smart-seq3 map splicing heterogeneity in brain tumor microenvironments, identifying therapy-resistant variants (e.g., EGFRvIII).
- Proteomic Validation: Mass spectrometry (DIA-MS) quantifies splice variant protein expression, confirming functional differences in TP53 isoforms (p53β/p53γ) during chemotherapy resistance.
3. Gene Editing and Functional Validation
- CRISPR-Splice: Targets splicing regulatory regions with sgRNAs to induce exon skipping or inclusion (e.g., restoring DMD reading frames in Duchenne muscular dystrophy).
- Minigene Reporters: Fluorescent constructs screen regulatory elements (e.g., SOD1 exon 2 mutations in ALS).
IV. Disease Mechanisms and Precision Medicine
1. Splicing Dysregulation in Disease
- Neurodegeneration: Alzheimer’s disease involves MAPT exon 10 retention, disrupting Tau protein 4R/3R ratios and driving neurofibrillary tangles.
- Cancer:
- CDKN2A exon 1β skipping produces oncogenic p14ARF truncations.
- MET exon 14 skipping activates pro-oncogenic signaling, emerging as a lung cancer therapeutic target.
- Rare Diseases: Spinal muscular atrophy (SMA) results from SMN1 exon 7 skipping, truncating SMN protein.
2. Diagnostic and Therapeutic Strategies
- Splicing Variant Classification: ACMG guidelines integrate SpliceAI predictions (PVS1 criteria) and RNA sequencing to improve pathogenicity assessment.
- Antisense Oligonucleotides (ASOs):
- Nusinersen (Spinraza®): Targets SMN2 exon 7 enhancers to boost full-length SMN protein in SMA.
- Eteplirsen (Exondys 51®): Induces DMD exon 51 skipping, partially restoring dystrophin function.
- Small-Molecule Modulators:
- Branaplam (LMI070): Stabilizes SMN2 pre-mRNA-spliceosome complexes to retain exon 6.
- H3B-8800: Inhibits SF3B1 to selectively kill splicing-deficient cancer cells (e.g., myelodysplastic syndromes).
V. Challenges and Future Directions
1. Overcoming Technical Barriers
- Model Limitations: Current tools (e.g., SpliceAI) struggle with distal regulatory elements (>100 bp). Graph neural networks (GNNs) integrating 3D genome (Hi-C) data may improve predictions.
- Clinical Translation:
- Liquid Biopsy: Combines ctRNA and single-cell profiling for non-invasive splicing analysis.
- Functional Annotation: Organoid models (e.g., brain organoids) validate splice variant pathogenicity.
2. Emerging Research Frontiers
- Synthetic Splicing Circuits: Engineered splicing factors (e.g., dCas9-SR/hnRNP fusions) program cell-specific splicing.
- Cross-Species Splicing Evolution: Comparative primate-rodent studies reveal human-specific disease mechanisms (e.g., schizophrenia-linked splicing).
- Splicing and Immunotherapy: Tumor neoantigen discovery focuses on splice-derived peptides (e.g., EGFRvIII) for personalized mRNA vaccines.
3. Industry and Clinical Integration
- AI-Driven Diagnostics: Platforms integrating SpliceTransformer and EHRs enable real-time clinical interpretation of splicing variants.
- Universal Splicing Therapies: LNP-delivered CRISPR-Cas13d systems (e.g., CasRx) degrade aberrant splice variants.
Conclusion
Alternative splicing underpins protein diversity and tissue specialization through spatiotemporal regulatory networks. Advances in deep learning, gene editing, and multi-omics integration are driving the field from mechanistic insights to clinical applications. Future breakthroughs in synthetic biology, AI-driven therapies, and dynamic regulatory modeling promise a “programmable splicing” era, transforming precision medicine and synthetic biology.
Data sourced from public references. Contact: chuanchuan810@gmail.com.