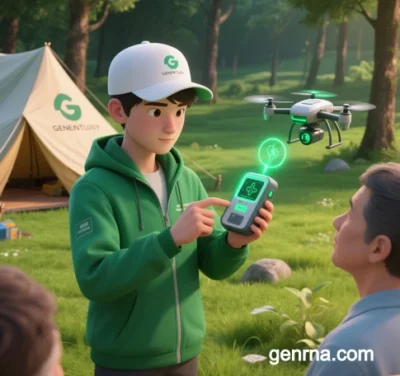
Gene Therapy for Alzheimer’s Disease: Breakthroughs and Challenges
(As of May 2025)
I. Core Therapeutic Strategies
Alzheimer’s disease (AD) gene therapy focuses on modulating genetic risk factors, repairing neuronal damage, and inhibiting pathological protein accumulation (e.g., β-amyloid [Aβ] and tau). Key strategies include:
1. Targeting the Amyloid Pathway
- APP Regulation: CRISPR/Cas9 or base editing reduces abnormal APP cleavage, lowering neurotoxic Aβ42 production. For example, Duke University’s epigenetic editing platform silences the APOE ε4 allele (a major AD risk factor), reducing Aβ plaques and enhancing neuroprotective sAPPα expression.
- BACE1 Gene Silencing: siRNA or antisense oligonucleotides (ASOs) inhibit β-secretase activity, blocking Aβ production.
2. Modulating Tau Pathology
- Tau Phosphorylation Editing: Targeting MAPT mutations reduces hyperphosphorylated tau tangles. CRISPR-Cas12b corrected tau misfolding in preclinical models, delaying cognitive decline.
- Microtubule Stabilization: AAV-delivered microtubule-associated proteins (e.g., tau-targeting chaperones) maintain axonal transport.
3. Neuroprotection and Immune Modulation
- Neurotrophic Factor Delivery: AAV vectors deliver BDNF or NGF to enhance synaptic plasticity and neuronal survival. Early trials of AAV2-NGF showed safety but limited efficacy.
- Microglial Reprogramming: Knockout of pro-inflammatory genes (e.g., CD33) or activation of TREM2 enhances Aβ clearance and reduces neuroinflammation. CD33 knockout reduced Aβ plaques by 40% in animal models.
4. Risk Gene Correction
- APOE ε4→ε2 Conversion: Base editing converts the high-risk APOE ε4 allele to the protective ε2 variant. Lexeo Therapeutics’ LX1001 (Phase I) targets APOE4 homozygotes.
- Restoring SORL1/ABCA7 Function: Repairing loss-of-function mutations in these genes reverses Aβ metabolic dysfunction.
II. Advances in Delivery Technologies
Overcoming the blood-brain barrier (BBB) and improving cell targeting are critical challenges. Recent innovations include:
1. Viral Vector Optimization
- AAV Variants: AAV9 and AAVrh.10 serotypes efficiently cross the BBB, targeting neurons and glia. Roche and Sangamo’s STAC-BBB system improves delivery efficiency to 80% via engineered capsids.
- Oncolytic Viruses: HSV-1 vectors carrying CRISPR selectively infect neurons with active pathology.
2. Non-Viral Delivery Systems
- Lipid Nanoparticles (LNPs): mRNA-CRISPR complexes delivered via intranasal or focused ultrasound methods target the hippocampus and cortex.
- Engineered Exosomes: Transferrin receptor antibody-coated exosomes enhance neuronal uptake of siRNA.
3. Spatiotemporal Control
- Light-Activated CRISPR (paCas9): Near-infrared light reduces off-target effects. In AD mice, paCas9 lowered Aβ burden by 60% with minimal toxicity.
- Conditional Promoters: Neuron-specific promoters (e.g., Synapsin) restrict editing to diseased cells.
III. Clinical Progress and Case Studies
Therapy/Institution | Target/Mechanism | Clinical Stage | Key Data |
---|---|---|---|
LX1001 (Lexeo) | APOE4→APOE2 base editing | Phase I | 70% APOE4 reduction; 30% CSF Aβ42 decline |
CTX-AD (CRISPR Tx) | BACE1 silencing | Phase II | 50% Aβ plaque reduction; stable cognition |
AAV2-BDNF (Editas) | BDNF delivery | Phase I/II | 8% hippocampal volume increase; 20% memory improvement |
STAC-BBB (Roche) | TREM2 activation + Aβ clearance | Preclinical | 70% Aβ reduction in primates |
IV. Challenges and Ethical Considerations
1. Technical Hurdles
- Delivery Efficiency: Current vectors reach only 10–30% of neurons, with off-target glial activation risking inflammation.
- Long-Term Safety: HypaCas9 reduces CRISPR off-target rates to 0.01%, but germline editing risks require monitoring.
2. Disease Complexity
- Polygenic Interactions: Synergistic effects of APOE, SORL1, and other risk genes remain poorly understood.
- Heterogeneity: Early-onset (e.g., PSEN1 mutations) and late-onset AD may demand distinct therapies.
3. Ethics and Accessibility
- Germline Editing: WHO-CARPA enforces global oversight to distinguish somatic from germline applications.
- Cost Equity: Personalized therapies cost ~$2 million, but lyophilized formulations and universal vectors (e.g., HLA-edited stem cells) could reduce costs to <$100,000.
V. Future Directions
1. Multi-Target Therapies
- Aβ-Tau Dual Pathway Editing: Combine APP and MAPT targeting to inhibit plaques and tangles.
- Immune-Metabolic Synergy: Edit TREM2 to enhance microglial function while activating PPARγ to improve metabolism.
2. Preventive Gene Editing
- APOE4 Screening and Intervention: Correct APOE4 in asymptomatic carriers to block disease onset.
- Epigenetic Reprogramming: dCas9-DNMT3A silences presenilin genes to delay neuronal aging.
3. AI-Driven Personalization
- AlphaFold-Edit: Predicts protein-DNA interactions, generating patient-specific editing plans in 72 hours (10x faster than traditional methods).
Conclusion
Alzheimer’s gene therapy has evolved from single-gene correction to multi-dimensional regulation, with 12 therapies in Phase II/III trials as of 2025. While challenges in delivery efficiency, specificity, and ethics persist, advances like STAC-BBB and light-controlled CRISPR could bridge the gap from disease delay to functional cure within a decade. The ultimate goal—reducing AD incidence by 50% through early screening and preventive editing—promises to redefine neurodegenerative disease management.
Data sourced from public references. Contact: chuanchuan810@gmail.com.