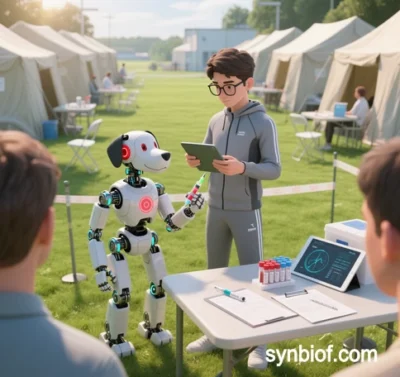
Synthetic Biology (SynBio): Engineering Biosystems for Medicine, Energy, and Materials
Synthetic biology (SynBio) is a revolutionary discipline that merges engineering principles with biological systems to design, construct, and optimize novel organisms or biological components. By reprogramming genetic circuits, metabolic pathways, and cellular behaviors, SynBio enables transformative applications across medicine, energy, materials, and beyond. Below is a comprehensive analysis of its core frameworks, applications, challenges, and future directions, synthesized from the provided evidence.
Core Frameworks of Synthetic Biology
- Redesigning Biological Systems
SynBio focuses on re-engineering organisms by modifying genetic codes, cellular processes, and metabolic networks. Key strategies include:- Standardized Biological Parts: Modular genetic components (e.g., promoters, ribosome-binding sites) enable predictable assembly of synthetic pathways .
- Computational Design: AI and machine learning (ML) optimize gene circuits, predict protein structures (e.g., AlphaFold), and simulate metabolic fluxes .
- DBTL Cycle: The “Design-Build-Test-Learn” framework accelerates iterative refinement of biological systems .
- Synthetic Organisms and Functions
- Artificial Life: Constructing organisms with non-natural functions, such as bacteria that degrade plastics or produce biofuels .
- Dynamic Control: CRISPR-based circuits and logic gates enable real-time regulation of gene expression to avoid metabolic overload .
Applications in Medicine
1. Drug Discovery and Production
- Target Identification: ML models analyze multi-omics data (genomics, proteomics) to predict drug targets and optimize metabolic pathways (e.g., artemisinin synthesis) .
- Cell Factories: Engineered microbes (e.g., E. coli) produce complex therapeutics like taxol precursors (18x yield increase) and CAR-T cell therapies (95% editing efficiency) .
- Antibiotic Development: Generative adversarial networks (GANs) design novel antibiotic scaffolds, reducing R&D cycles from 5–10 years to 6 months .
2. Precision Therapeutics
- Gene and Cell Therapies: Synthetic gene circuits enable programmable therapies, such as optogenetic tools for Parkinson’s disease and suicide switches (HSV-TK) to eliminate aberrant cells .
- Biosensors: CRISPR-Cas systems with ML-optimized gRNA detect pathogens (e.g., COVID-19 variants) at 1 copy/μL, while logic-gated circuits distinguish cancer cells (>99% specificity) .
3. Diagnostic Tools
- Wearable Biochips: Engineered bacteria monitor biomarkers (e.g., IL-6) in real time, transmitting data via Bluetooth .
- Early Cancer Screening: ctDNA sequencing combined with ML models predicts tumor risk with AUC 0.93 .
Energy and Sustainability
- Biofuel Production
- Metabolic Engineering: Optimized pathways in yeast or algae produce ethanol, butanol, and biodiesel. For example, Saccharomyces cerevisiae engineered for β-carotene synthesis achieves high yields .
- Carbon Capture: Synthetic bacteria fix CO₂ 50x faster than algae, converting it into biofuels or biodegradable plastics .
- Circular Economy
- Waste Upcycling: Microbial consortia convert plastic waste into polyhydroxyalkanoates (PHA) with >90% efficiency .
- Sustainable Raw Materials: Vanillin and bio-leather are produced from non-petrochemical sources, reducing environmental impact .
Materials Innovation
- Biodegradable Polymers
- Polylactic Acid (PLA): Engineered Bacillus subtilis strains synthesize PLA from renewable feedstocks, replacing petroleum-based plastics .
- Spider Silk: Recombinant proteins produced in yeast or bacteria yield high-performance fibers for textiles and medical sutures .
- Advanced Biomaterials
- Self-Healing Materials: Synthetic biofilms with dynamic gene circuits repair cracks autonomously .
- Smart Hydrogels: Environmentally responsive polymers release drugs or growth factors in response to pH or temperature changes .
Challenges and Ethical Considerations
- Technical Barriers
- Scalability: High costs and low efficiency in large-scale production hinder commercialization (e.g., synthetic meat) .
- Predictability: Unintended interactions in complex genetic networks remain a challenge .
- Ethical and Safety Issues
- Biosafety: CRISPR molecular tags track synthetic organisms to prevent unintended environmental release .
- Equity: Open-source platforms (e.g., Open Haptics Alliance) democratize access to SynBio tools, addressing patent barriers and demographic biases .
Future Directions
- Convergence with AI and Quantum Computing
- AI-Driven Design: Generative models predict novel metabolic pathways and optimize genetic circuits .
- Quantum Simulations: IBM quantum processors accelerate protein folding predictions 100x, enabling rapid enzyme design .
- Next-Generation Applications
- Regenerative Medicine: Synthetic tissues and organs with programmable growth factors .
- Space Biomanufacturing: Engineered microbes produce oxygen and nutrients during long-term space missions .
- Policy and Governance
- Global Standards: Harmonized regulations for synthetic organism containment and IP management .
Conclusion
Synthetic biology is reshaping industries by bridging biology and engineering. In medicine, it enables personalized therapies and rapid diagnostics; in energy, it drives sustainable fuel production; and in materials, it pioneers eco-friendly alternatives. While challenges like scalability and ethics persist, advancements in AI, automation, and interdisciplinary collaboration promise a future where SynBio solutions are both transformative and accessible. As the field matures, its potential to address global crises—from climate change to pandemics—will solidify its role as a cornerstone of 21st-century innovation.