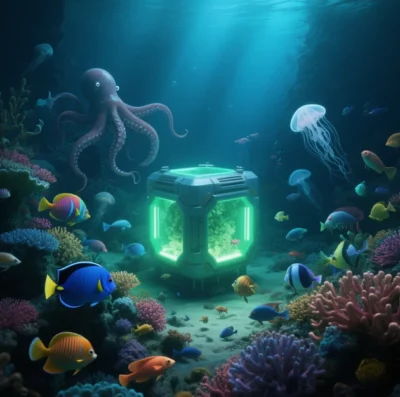
Challenges and Future Directions of CRISPR Technology
Despite CRISPR’s revolutionary potential in precision medicine and gene therapy, its large-scale clinical application faces multiple challenges. Below, we outline key technical bottlenecks and future directions, integrating current research progress and ethical considerations.
Key Challenges
Delivery Efficiency and Tissue Specificity
- Delivery Vector Limitations: Viral vectors (e.g., AAV) target specific tissues (e.g., liver) but face immunogenicity and cargo capacity constraints. Non-viral vectors (e.g., lipid nanoparticles, LNPs) struggle with penetration in solid tumors, achieving only ~5% editing efficiency.
- Tumor Microenvironment Barriers: Dense extracellular matrices and immunosuppressive microenvironments in solid tumors hinder CRISPR delivery, necessitating smart strategies like exosome- or pH-responsive nanoparticles.
Off-Target Effects and Safety
- Non-Specific Editing Risks: Traditional Cas9 exhibits off-target rates of 0.1-5%, potentially activating proto-oncogenes or disrupting tumor suppressors. Early trials reported safety concerns, such as T-cell chromosomal translocations.
- High-Fidelity Tools: HypaCas9 and Cas12a reduce off-target rates by 10-100x. Base editors (e.g., ABE, CBE) avoid double-strand breaks, further mitigating risks.
Ethical and Equity Concerns
- Gene Drive Risks: Engineered microbes (e.g., carbon-capturing cyanobacteria) require dual biocontainment (e.g., temperature-sensitive kill switches + nutrient dependency) to prevent ecological disruption.
- Therapeutic Accessibility: Most CRISPR therapies rely on European genomic data, neglecting genetic diversity in African and other minority populations (e.g., APOL1 nephropathy), exacerbating health inequities.
Tumor Heterogeneity and Drug Resistance
- Clonal Evolution: Dynamic genomic heterogeneity in tumors undermines single-editing strategies. For example, EGFR T790M mutations drive resistance to osimertinib, requiring CRISPR-guided combination therapies.
Immunogenicity and Long-Term Safety
- Anti-Cas9 Immunity: ~30% of populations have pre-existing anti-Cas9 antibodies, risking inflammation or reduced efficacy. Solutions include humanized Cas variants (e.g., SaCas9-KKH) or transient mRNA delivery.
Future Breakthrough Directions
AI-Driven Precision Design
- sgRNA Optimization: AlphaFold for RNA predicts sgRNA secondary structures, while deep learning models (e.g., DeepCRISPR) reduce off-target rates below 0.01%.
- Patient-Specific Therapies: Tumor mutation profiles guide personalized sgRNA libraries (e.g., logic-gated CAR-T cells targeting KRAS G12D or TP53 mutations).
In Vivo Editing and Epigenetic Regulation
- Systemic Delivery: Inhalable LNPs deliver CRISPR to the lungs for cystic fibrosis; blood-brain barrier-penetrating nanoparticles (e.g., Angiopep-2-modified) edit Huntington’s disease genes.
- Epigenome Editing: CRISPR-dCas9 fused with epigenetic modifiers (e.g., DNMT3A, HDAC) silences oncogenes without altering DNA sequences.
Universal Therapies and Scalable Production
- Off-the-Shelf Cell Therapies: Knockout of HLA-I genes (e.g., B2M) and immune checkpoints (e.g., PD-1) enables allogeneic CAR-T/NK cells, slashing costs to 1/10 of traditional therapies.
- Automated Biofoundries: AI-controlled microfluidic platforms enable parallel editing of thousands of cell lines, accelerating drug target discovery via CRISPR libraries.
Next-Gen Editors and Multi-Omics Integration
- Prime Editing: Enables precise insertions/deletions without double-strand breaks, achieving 45% efficiency in sickle cell mouse models.
- Quantum-Bio Hybrids: Quantum computing simulates codon-anticodon interactions to engineer unnatural base pairs (e.g., dNaM-dTPT3), expanding genetic data storage.
Global Collaboration and Ethical Governance
- Data Sharing Platforms: Databases like CRISPRme standardize off-target assessments and promote international clinical trial data sharing.
- Dynamic Regulation: The EU’s SynBio-Safety Level (SBSL) framework requires global coordination with WHO to ensure equitable CRISPR therapy access.
Roadmap and Milestones
Timeline | Key Goals |
---|---|
2025-2027 | – High-fidelity base editors (e.g., VERVE-101) complete Phase III trials for hereditary hypercholesterolemia. – AI-optimized delivery systems achieve 20% editing efficiency in solid tumors. |
2028-2030 | – Universal CAR-T therapy costs drop to $50,000 per course, covering >50% of B-cell lymphoma patients. – Quantum-CRISPR platforms validate unnatural base editing in vitro. |
2031-2035 | – Epigenetic editing therapies approved for breast and colorectal cancers, raising 5-year survival to 80%. – Global CRISPR networks expand to low-income regions, improving equity by 30%. |
Conclusion: From Technical Breakthroughs to Ecological Responsibility
CRISPR is evolving from a “gene-editing tool” to a “life-programming platform,” shifting focus from technical bottlenecks to systemic risk management. Over the next decade, interdisciplinary collaboration (AI, quantum computing, synthetic biology) and global governance could drive three transformative leaps:
- Precision Leap: From base editing to epigenome programming, enabling full control of biological information flow.
- Accessibility Leap: From costly therapies to affordable healthcare, breaking geographic and economic barriers.
- Ethical Leap: From anthropocentrism to ecological synergy, balancing innovation with biosafety.
Ultimately, CRISPR will transcend disease treatment to become a universal “ecological design language” for reshaping health paradigms.
Data sourced from public references. For collaboration or domain inquiries, contact: chuanchuan810@gmail.com