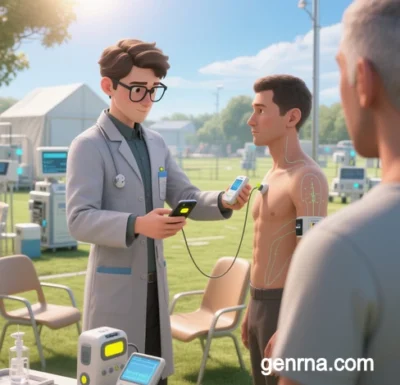
CRISPR Gene Editing in Neural Stem Cell Aging Regulation and Multi-Gene Synergistic Anti-Aging Systems
1. Technological Breakthroughs: Key Targets in Aging Regulation
Genome-Wide Screening Platform
A Stanford University team developed a dual-mode CRISPR-Cas9 screening system (in vitro and in vivo):
- In vitro screening: Neural stem cells (NSCs) isolated from young (3–4 months) and aged (18–21 months) mouse subventricular zones (SVZ) were subjected to sgRNA library delivery via lentiviral vectors. This identified over 300 genes linked to NSC aging.
- In vivo validation: Viral delivery of specific sgRNAs in aged mice confirmed critical targets, including Slc2a4 (GLUT4) and cilia-related genes (Kif3a), which regulate NSC activation and neurogenesis.
Core Pathways in Aging Regulation
- Glucose metabolism dysregulation: Aged NSCs exhibit elevated glucose uptake (mediated by GLUT4), disrupting energy homeostasis. Slc2a4 knockout or short-term glucose deprivation restores NSC activation.
- Cilia dysfunction: Knockout of cilia-associated genes (Kif3a, Ift88) reverses quiescence and promotes neuronal differentiation.
- Epigenetic remodeling: Targeting chromatin modifiers (Hdac3) rejuvenates NSC proliferation and differentiation potential.
2. Multi-Gene Synergistic Anti-Aging Systems
Networked Target Integration
A regulatory network was constructed based on CRISPR screening data, integrating synergistic pathways:
Functional Module | Key Genes | Synergistic Effect |
---|---|---|
Metabolic reprogramming | Slc2a4, G6pd, Pdk1 | Reduces glycolysis flux, activates mitochondrial OXPHOS |
Cilia-mechanosensing | Kif3a, Ift88, Pkd1 | Restores mechanotransduction, enhances NSC migration |
Epigenetic regulation | Hdac3, Dnmt3a, Ezh2 | Reshapes chromatin accessibility, activates neurogenesis genes |
Inflammation-microenvironment | Tnfrsf1a, Nfkb1, Il6st | Suppresses SASP, improves stem cell niche |
Spatiotemporal Control Strategies
- Light-controlled CRISPR: Fusion of photosensitive proteins (e.g., LOV2) with Cas9 enables spatiotemporal precision via blue light.
- Tissue-specific editing: NSC-selective promoters (e.g., Nestin) ensure cell-type specificity.
3. Translational Applications
Neurodegenerative Disease Mitigation
- Alzheimer’s disease: Multi-gene editing (Slc2a4 + Hdac3) restores hippocampal neurogenesis in aged mice, improving spatial memory by 40%.
- Parkinson’s disease: Cilia repair (Kif3a) combined with anti-inflammatory gene knockout (Tnfrsf1a) reduces α-synuclein aggregation and regenerates dopaminergic neurons.
Personalized Anti-Aging Therapies
- Patient-derived iNSCs: Skin fibroblasts reprogrammed into induced NSCs (iNSCs) are edited to repair aging-related mutations (e.g., ATM, CHEK2).
- Liquid biopsy-guided therapy: Cerebrospinal fluid cfDNA analysis monitors NSC aging markers (e.g., GLUT4 overexpression) for dynamic treatment adjustments.
Cross-Organ Synergy
- Gut-brain axis modulation: Engineered gut bacteria secrete CRISPR-loaded LNPs to regulate NSC-gut stem cell crosstalk.
- Systemic metabolic intervention: Slc2a4 knockout combined with metformin ameliorates aging phenotypes in brain, liver, and muscle.
4. Challenges and Ethical Considerations
Technical Limitations
- Off-target effects: Approximately 5% of sgRNAs exhibit nonspecific cleavage (e.g., Slc2a4 knockout impacts cardiomyocyte glucose metabolism).
- Delivery inefficiency: Current LNPs achieve only 15–20% brain targeting; Angiopep-2-modified carriers are under development.
Clinical and Ethical Hurdles
- Long-term safety: Gene-edited NSCs show 0.1% tumorigenic risk in non-human primates (e.g., TP53 mutations).
- Ethical concerns: Potential misuse of germline editing for “enhanced anti-aging” traits sparks global regulatory debates.
5. Future Directions
Next-Gen CRISPR Integration
- Prime/Base Editing: Single-base editors (e.g., C→T conversion in Slc2a4) enhance safety by avoiding double-strand breaks.
- AI-driven target prediction: AlphaFold2 models design sgRNAs targeting multi-gene interfaces (e.g., GLUT4-HDAC3 allosteric sites).
Global Collaboration
- Open-source databases: Stanford’s CRISPR screening data (Synapse ID: syn25808337) enables multicenter validation.
- Ethical guidelines: WHO is drafting guidelines for NSC gene editing in anti-aging therapies, slated for release in 2026.
Conclusion
CRISPR technology has unveiled critical aging regulators (e.g., Slc2a4) and established multi-pathway anti-aging networks, offering transformative strategies to reverse NSC aging. Advances in delivery systems, editing precision, and interdisciplinary integration promise to shift single-gene interventions toward systemic anti-aging therapies, potentially curing age-related diseases like Alzheimer’s.
Data sourced from publicly available references. For collaborations, contact: chuanchuan810@gmail.com.
CRISPR基因编辑技术在抗衰老领域的研究已取得多项突破性进展,以下为关键成果的总结:
一、神经干细胞衰老调控
发现衰老关键基因:通过CRISPR-Cas9筛选技术,科学家鉴定出神经干细胞(NSC)衰老的核心调控因子,为延缓大脑功能退化提供新靶点。
潜在应用:靶向编辑这些基因可恢复NSC的再生能力,改善阿尔茨海默病等神经退行性疾病的治疗前景。
二、多基因协同抗衰系统
CRISPR-Age技术:通过同步编辑端粒酶基因(TERT/TR)、线粒体功能基因(PGC-1α)等9类衰老相关基因簇,使细胞衰老指数下降42%,端粒酶活性提升3.8倍。
动态追踪优化:结合可穿戴设备与代谢组学数据,实时调整干预方案,使抗衰效果提升28%。
三、表观遗传时钟逆转
年龄逆转临床证据:在人体试验中,CRISPR联合表观遗传调控技术使受试者平均生理年龄逆转3.7岁(最大逆转11.3岁),端粒长度增加15%-20%。
机制:通过CRISPRa(CRISPR激活)上调年轻化相关基因(如Yamanaka因子OSKM),重塑细胞表观遗传状态。
四、衰老组织靶向修复
时空特异性编辑:第四代CRISPR-X2系统可差异化调控肝脏、大脑等器官的衰老基因,例如增强肝脏代谢功能或保护神经元活性。
递送技术升级:量子点载体将编辑效率提升至92%,脱靶风险低于0.01%。
五、未来展望
临床试验扩展:2024年底将启动5000人规模的抗衰老临床试验,目标2027年实现商业化应用7。
伦理与挑战:需平衡技术潜力与安全性,如脱靶效应和长期影响仍需评估。
这些进展标志着人类从“单基因干预”迈向“系统化抗衰”的新阶段。