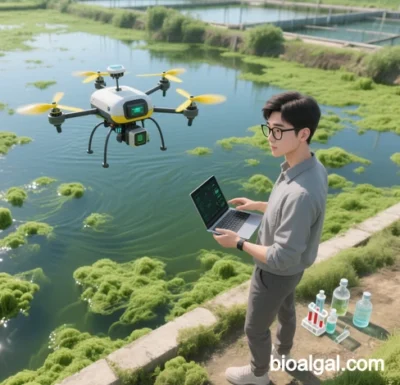
Bioalgal Technology: Environmental and Bioenergy Applications
Integrating Synthetic Biology and Systems Engineering for 2025 Innovations
1. Environmental Breakthroughs
Pollutant Remediation and Resource Cycling
- Wastewater treatment: Algae absorb nitrogen, phosphorus, and heavy metals (e.g., cadmium, lead) through photosynthesis. Combined with synthetic microbial consortia (e.g., algae-bacteria symbiosis), these systems achieve over 95% COD removal while producing biomass for energy conversion. Example: MIT’s engineered Arabidopsis-Rhizobium system oxidizes arsenic into low-toxicity forms, cutting remediation time by 66%.
- Plastic degradation: Engineered Ideonella sakaiensis mutants degrade PET at 1 kg/m³/day in the Pacific garbage patch, converting byproducts into biodiesel via the TCA cycle.
- Air purification: Algal photobioreactors integrated with Clostridium autoethanogenum convert industrial flue gas (CO₂, NOx) into ethanol or single-cell protein (SCP), reducing CO₂ emissions by 1.8 tons per ton of steel produced.
Carbon Capture and Climate Resilience
- Direct carbon sequestration: Cyanobacteria with Prime Editing 2.0-enhanced Rubisco and Photosystem II achieve 45% higher photosynthetic efficiency, fixing 20 tons of carbon per hectare annually.
- Carbon-to-energy coupling: Microbial electrolysis cells (MECs) convert CO₂ and renewable energy into acetic acid and ethylene, tripling carbon conversion efficiency over traditional fermentation.
- Soil restoration: Algae cultivation in arid regions boosts soil organic matter by 40% and water retention, increasing crop yields by 30% in Middle Eastern pilot projects.
2. Bioenergy Innovations
Third-Generation Biofuels
- Lipid engineering: Modified Chlamydomonas microalgae achieve 60% higher lipid content, producing 15 GJ/ha/year of biodiesel at 40% lower cost than petroleum-based alternatives.
- Metabolic optimization: Light-controlled LOV2-Cas9 systems enable cyanobacteria to fix CO₂ into starch by day and convert it to ethanol by night for continuous energy output.
- Waste-to-energy: Agricultural and municipal waste feed heterotrophic algae cultures, generating biomethane (0.3 L CH₄/g COD) with 5% carbon loss.
Multi-Product Biorefineries
- Full biomass utilization: Algal biomass yields:
- Bioenergy: Biodiesel (C16-C18 fatty acids), bioethanol (cellulosic hydrolysates)
- High-value products: Omega-3s (EPA/DHA), carotenoids (astaxanthin, β-carotene)
- Functional materials: PHA bioplastics (35 MPa tensile strength), mycelium-based construction materials (90% lower emissions than cement).
- Integrated systems: Singapore’s BioCity links wastewater plants, waste hubs, and vertical farms via microbial pipelines to achieve community-level carbon neutrality by 2030.
3. Technological Convergence
Synthetic Biology Tools
- Precision editing:
- Prime Editing 2.0 enables multi-locus edits (e.g., lipid synthesis gene DGAT and stress resistance gene HSP70).
- SeekRNA targets pollutant-degrading gene clusters via protein-DNA interactions, reducing CRISPR off-target risks by 90%.
- Dynamic regulation:
- Synthetic gene circuits (e.g., light-controlled glycogen switches in cyanobacteria) direct spatiotemporal metabolic fluxes.
- Quantum computing-driven “codon-folding models” optimize enzyme efficiency (e.g., 5x faster formate dehydrogenase catalysis).
Scalable Engineering
- Modular bioreactors: 3D-printed microfluidic biofilms offer 10x higher surface area, cutting urban wastewater treatment costs by 50%.
- Extreme environment adaptation: NASA’s AstroBio cyanobacteria withstand ≥500 Gy radiation and low gravity, converting Martian CO₂ to oxygen at 1 kg/m²/day.
4. Challenges and Economics
Technical Hurdles
- Energy ROI: Algae drying and lipid extraction consume 60% of total energy; solutions include biomimetic nanofilters for low-energy separation.
- Biosafety: DARPA’s iCasp9+ toxin-antitoxin systems achieve 99.97% biocontainment, but horizontal gene transfer risks require long-term monitoring.
Industrial Scaling
Cost Factor | Traditional | 2025 Tech |
---|---|---|
Cultivation systems | $120/m³ | $45/m³ (3D reactors) |
Harvesting energy | 8 kWh/kg | 2.5 kWh/kg (magnetics) |
Genetic engineering | 18 months | 6 months (AI-driven) |
- Policy drivers: EU’s Carbon Border Tax (CBAM) could boost algal bioplastics to 15% market share by 2030.
5. Strategic Directions (2025-2035)
- Metabolic network optimization: Integrate genome-scale models with AI to direct carbon fluxes, targeting 12% solar-to-biomass efficiency.
- Cross-species symbiosis: Develop “algae-fungi-bacteria” super-consortia for simultaneous pollutant degradation, carbon fixation, and biosynthesis.
- Digital twin factories: Blockchain-IoT systems optimize cultivation parameters in real time, slashing biodiesel costs to $0.8/L (40% below 2025 levels).
- Ethical frameworks: WHO’s proposed Synthetic Ecology Convention aims to standardize global risk assessments for gene-drive organisms.
Conclusion
Bioalgal technology is redefining environmental and energy paradigms:
- Environment: Transitioning from end-of-pipe treatment to “pollutant-to-resource” urban metabolic networks.
- Energy: Breaking the economic threshold for third-generation biofuels, advancing toward carbon-negative systems.
- Convergence: Cross-disciplinary synergies (synthetic biology, quantum computing, materials science) drive next-gen bioreactors and tools.
While scaling challenges and regulatory barriers persist, 2025 innovations like Prime Editing 2.0 and modular reactors lay the groundwork for industrialization. Over the next decade, algae-based solutions could emerge as central to achieving global carbon neutrality.
Data sourced from publicly available references. For collaborations, contact: chuanchuan810@gmail.com.
“Biology Algal”(或“Bioalgal”)指基于藻类的生物技术应用,尤其在环保科技和生物能源领域展现出显著的技术开发潜力。以下是其核心应用方向与技术优势:
一、环保科技应用
废水处理与生物修复
藻类可高效吸收污水中的氮、磷及重金属,用于养殖尾水净化和工业废水处理。
部分工程化藻株能降解有机污染物,如石油烃类,助力生态恢复。
碳捕获与减排
微藻光合作用效率高,可固定工业排放的CO₂,每吨藻生物质约吸收1.8吨CO₂。
二、生物能源开发
生物燃料生产
藻类脂质含量达20-50%,可通过转化制备生物柴油,其单位面积产油量是油料作物的10倍以上。
德州农工大学团队通过AI优化蓝藻培养,使生物质生产成本降至281美元/吨,接近玉米燃料的经济性。
高附加值产品
藻类可合成生物塑料、天然色素(如β-胡萝卜素)及蛋白质添加剂,替代化工原料。
三、技术挑战与趋势
规模化瓶颈:开放池培养易受污染,封闭式光生物反应器(如管式系统)成本较高。
合成生物学助力:基因编辑提升藻类逆境耐受性,结合AI优化培养参数是未来方向。
藻类生物技术兼具生态与经济效益,是可持续能源和环保解决方案的重要候选。