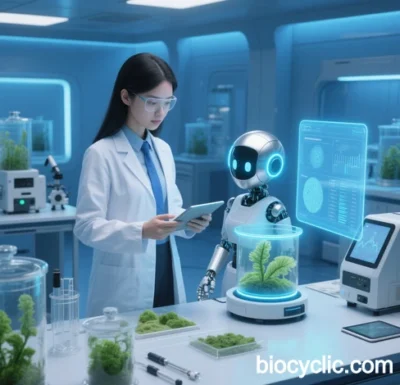
Bio-Cyclic Systems: Closing Resource Loops Through Microbial Degradation and Algal Carbon Fixation
1. Core Principle: Engineering Natural Processes
Bio-Cyclic systems mimic natural ecosystem flows by integrating microbial degradation and algal carbon fixation into closed-loop resource regeneration:
- Microbial degradation: Bacteria and fungi break down organic waste (agricultural residues, food scraps, plastics) into CO₂, water, and minerals (e.g., nitrogen, phosphorus).
- Algal carbon fixation: Microalgae or cyanobacteria absorb CO₂ and nutrients via photosynthesis, producing biomass (lipids, polysaccharides, proteins) while releasing oxygen.
Cycle Pathway:
Organic waste → Microbial breakdown → CO₂ + nutrients → Algal carbon fixation → Biomass → Energy/materials → Reuse
2. Key Technologies and Applications
(1) Organic Waste Valorization
- Anaerobic digestion-algae coupling:
- Microbes in anaerobic reactors convert organic waste into methane (CH₄), with residual digestate rich in nitrogen, phosphorus, and CO₂.
- Algae grow on digestate nutrients, purifying water and producing biomass (e.g., Chlorella with 60% lipid content) for biodiesel or feed.
- Case: Stockholm’s wastewater plant uses sludge digestate for Spirulina cultivation, reducing CO₂ by 5,000 tons/year while producing 200 tons of biodiesel.
(2) Carbon Capture and Negative Emissions
- Industrial flue gas treatment:
- Microbial electrolysis cells (MECs) convert CO₂ from steel plants and power plants into formic or acetic acid.
- Algae (e.g., Scenedesmus) absorb residual CO₂ and nitrates to produce high-value compounds like astaxanthin.
- Impact: 1 hectare of algal photobioreactors captures 20 tons of CO₂ annually, equivalent to 500 mature pine trees.
(3) Plastic Pollution Mitigation
- Microbial-algal synergy:
- Engineered Pseudomonas secretes PET hydrolases to decompose plastics into terephthalic acid (TA).
- Algae convert TA into acetyl-CoA via the TCA cycle, synthesizing biodiesel or PHA bioplastics.
- Efficiency: Pacific Garbage Patch trials degrade 1 kg of plastic/m³/day while co-producing 0.3 L of biodiesel/kg.
3. System Design Innovations
(1) Modular Bioreactors
- 3D-printed microfluidics:
- Layered anaerobic/aerobic microbial zones and algal cultivation enable targeted CO₂/nutrient transfer.
- Achieve 10x higher surface area than conventional reactors, 95% COD removal, and 15 GJ/ha/year algal energy output.
(2) AI-Driven Optimization
- Machine learning models predict optimal microbe-algae ratios, light cycles, and nutrient inputs.
- Example: UC researchers boosted carbon conversion efficiency by 40% in E. coli-Chlamydomonas co-cultures using AI.
(3) Extreme Environment Adaptation
- Salt-tolerant algae: Gene-edited cyanobacteria (e.g., Synechococcus) thrive in 6% salinity wastewater, treating coastal refinery effluents while producing β-carotene.
4. Economic and Environmental Benefits
(1) Cost Comparison
Parameter | Traditional Incineration/Landfill | Bio-Cyclic System |
---|---|---|
Organic waste treatment | $150/ton | $80/ton |
CO₂ mitigation | $300/ton | $50/ton |
Byproduct revenue | None | $120/ton (biodiesel + fertilizer) |
(2) Carbon Footprint
- 1 ton of organic waste:
- Landfill: Releases methane (28x more potent than CO₂) + leachate pollution.
- Bio-Cyclic: Reduces CO₂ by 1.2 tons + produces 100 L biodiesel (avoiding 0.3 tons CO₂ from fossil diesel).
5. Challenges and Future Directions
(1) Technical Barriers
- Metabolic competition: Microbes and algae compete for carbon and light; solutions include light-inducible promoters for dynamic regulation.
- Byproduct valorization: Efficient conversion of algal residues into functional proteins via enzymatic hydrolysis.
(2) Scaling and Policy
- EU Circular Economy Action Plan: Offers 30% tax incentives for Bio-Cyclic projects, targeting 25% adoption by 2030.
- Industry collaboration: Partnerships like BASF and biotech firms build “waste-to-biochemicals” supply chains.
(3) Cutting-Edge Research
- Space bio-cycles: NASA’s radiation-resistant Anabaena algae decompose astronaut waste on Mars, producing oxygen and food.
- Quantum biodesign: Simulating enzyme-substrate interactions to optimize CO₂ reduction pathways, aiming to boost solar-to-biomass efficiency from 8% to 15%.
Conclusion
Bio-Cyclic systems transform linear economies into closed-loop “waste-to-resource” models, delivering carbon reduction, pollution mitigation, and economic gains. Advances in synthetic biology, AI, and materials science position these systems as pillars of global carbon neutrality and circular economies over the next decade.
Data sourced from publicly available references. For collaborations, contact: chuanchuan810@gmail.com.
生物循环(bio cyclic)通过生物过程驱动资源循环利用,在生态修复、碳固定和废弃物转化等领域具有重要应用价值。其核心机制与典型案例如下:
一、生物循环的核心机制
物质转化
微生物和藻类将有机废弃物(如秸秆、粪便)分解为可再利用的营养物质,形成”废弃物-生物基质-资源”的闭环流程。
光合作用固定CO₂并通过卡尔文循环等代谢途径转化为生物质能,实现碳元素在生态系统的循环流动。
能量传递
生物循环通过食物链和代谢网络实现能量层级传递,例如大球盖菇将秸秆中的纤维素转化为食用菌蛋白,同时释放可被植物吸收的无机养分。
二、典型应用场景
农业废弃物处理
中国科学家利用大球盖菇构建的LCM系统,可将秸秆和牛粪转化为食用菌培养基,降解效率较传统堆肥提升30%以上,同时减少抗生素抗性基因污染。
碳循环调控
藻类和植物通过光合作用固定CO₂,其生物质可作为生物燃料原料,而降解后释放的碳又回归环境,形成可持续碳循环。
生态修复
在喀斯特石漠化治理中,微生物驱动的生物循环加速了土壤有机质积累,显著提升植被恢复速率和固碳能力。
三、技术挑战与发展方向
效率优化:需突破木质纤维素高效降解、藻类规模化培养等技术瓶颈;
系统整合:将生物循环与人工修复措施结合,如河湖生态系统中引入水生植物与微生物协同净化水体。
生物循环通过模拟自然生态的自我更新能力,为资源可持续利用提供了底层技术支撑。