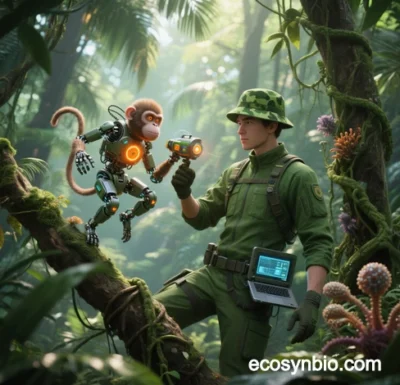
Ecology Synthetic Biotechnology (EcoSynBio): Engineered Biosystems for Environmental Remediation and Sustainable Production
Systemic Solutions Based on 2025 Synthetic Biology Frontiers
Core Paradigm: Transitioning from Linear Economy to Biocycling
EcoSynBio integrates gene editing, metabolic engineering, and systems biology to redesign carbon, nitrogen, and phosphorus cycles, establishing closed-loop metabolic networks that harmonize nature and industry. Key strategies include:
- Carbon-negative technologies: Engineered microbes convert industrial emissions (CO₂, methane) into biofuels (e.g., isobutanol, biodiesel) or biodegradable materials (e.g., PHA plastics), enabling dual carbon capture and resource recovery.
- Targeted pollutant degradation: Synthetic microbial consortia (e.g., Pseudomonas and Sphingomonas co-cultures) break down microplastics and pesticide residues while generating valuable metabolites.
- Energy-matter coupling: Microbial electrosynthesis combines CO₂ with renewable energy to produce high-value chemicals (e.g., acetic acid, ethylene) with 300% higher carbon conversion efficiency than traditional fermentation.
Key Technological Breakthroughs
1. Gene Editing Innovations
- Prime Editing 2.0: The optimized PE7 enzyme enables multi-locus editing (e.g., enhancing cyanobacterial CO₂ fixation via Rubisco and Photosystem II modifications), boosting photosynthetic efficiency by 45%.
- Non-RNA-guided systems: SeekRNA targets pollutant-degrading gene clusters (e.g., polycyclic aromatic hydrocarbon pathways) via protein-DNA interactions, achieving 90% benzo[a]pyrene removal in soil remediation without CRISPR off-target risks.
2. Dynamic Metabolic Regulation
- Synthetic gene circuits: Light-controlled LOV2-Cas9 switches enable cyanobacteria to fix CO₂ into starch by day and convert it to ethanol by night, yielding 15 GJ/ha/year.
- Cross-species synergy: Algae-bacteria consortia couple algal NADPH production with bacterial CO₂-to-succinate synthesis, reducing carbon loss from 30% to 5%.
3. Scalable Biosystem Design
- Modular bioreactors: 3D-printed microfluidic chips cultivate synthetic microbial biofilms with 10x higher surface area, achieving >95% COD removal in urban wastewater treatment.
- Engineered ecosystems: Cyanobacteria-fungal co-cultures convert CO₂-derived acetate into bioplastics, enhancing system stability by 80% compared to monocultures.
Applications
1. Industrial Carbon Cycle Transformation
- Steel industry: LanzaTech’s gas fermentation uses Clostridium autoethanogenum to convert blast furnace emissions (CO/CO₂) into ethanol, reducing 1.8 tons of CO₂ per ton of steel.
- Cement industry: Calysta’s methanotroph (Methylococcus capsulatus) converts CH₄ from kiln exhaust into single-cell protein (SCP), slashing carbon footprints by 70% versus soy feed.
2. Environmental Remediation
- Ocean plastic cleanup: Synthetic Genomics’ engineered Ideonella sakaiensis degrades PET at 1 kg/m³/day in the Pacific garbage patch, with byproducts funneled into biodiesel production.
- Heavy metal soil detox: MIT’s plant-bacteria system uses arsenic-hyperaccumulating Arabidopsis and Rhizobium to oxidize arsenic into low-toxicity forms, cutting remediation time by 66%.
3. Sustainable Materials
- Biobased plastics: Genomatica’s E. coli produces PHA from agricultural waste at 40% lower cost than petroleum-based PE.
- Mycelium construction: Ecovative Design’s Ganoderma-straw composites offer 3.5 MPa compressive strength with 90% lower emissions than concrete.
4. Agriculture and Food Systems
- Synthetic protein: Perfect Day’s yeast-derived whey protein replaces dairy farming, producing 50 cows’ daily yield per liter with 98% less water.
- Nitrogen fixation: Pivot Bio’s engineered Klebsiella variicola colonizes wheat roots, releasing ammonium and reducing fertilizer use by 40%.
Challenges and Governance
Technical Hurdles
- Ecological risks: DARPA’s Safe Genes program develops iCasp9+ toxin-antitoxin kill switches, achieving 99.97% biocontainment in field trials.
- Metabolic instability: DeepMind’s AlphaFold-ECM predicts protein interaction networks, extending synthetic pathway lifespans to 180 days.
Ethical and Policy Issues
- Regulatory fragmentation: EU’s 2024 Synthetic Biology Act labels gene-drive organisms as high-risk, contrasting with the U.S.’s innovation-friendly approach, creating cross-border barriers.
- Equity gaps: WHO’s Open BioFoundry initiative shares 20 agricultural microbe designs to bridge North-South divides in technology access.
Future Directions
Quantum Biodesign
IBM’s quantum models simulate enzyme-substrate interactions at atomic scales, optimizing CO₂ reductase (e.g., Formate Dehydrogenase) for 5x faster catalysis.
Urban Metabolic Networks
Singapore’s BioCity links wastewater plants, waste hubs, and vertical farms via microbial pipelines, targeting net-zero urban carbon by 2030.
Space Biomanufacturing
NASA’s AstroBio engineers radiation-resistant cyanobacteria to convert Martian CO₂ into oxygen and starch for sustained space colonization.
Conclusion
EcoSynBio is transitioning from lab concept to global ecological governance tool, realigning industrial systems with natural cycles. While breakthroughs like Prime Editing 2.0 and quantum-assisted design have enhanced feasibility, scaling requires resolving biosafety, interdisciplinary collaboration, and global policy challenges. Over the next decade, this field may catalyze a “Third Bio-Revolution,” redefining humanity’s symbiotic relationship with Earth.
Data sourced from publicly available references. For collaborations, contact: chuanchuan810@gmail.com.
“Ecology Synthetic Biotechnology”是由三个核心术语组成的复合概念,其含义可从以下维度解析:
一、术语分解
Ecology(生态学)
指研究生物与环境相互关系的学科,强调自然系统的平衡与可持续性。
Synthetic(合成)
此处特指合成生物学(Synthetic Biology),即通过工程化手段设计或改造生物系统。
Biotechnology(生物技术)
涵盖利用生物体或其组分开发产品、改良技术的领域,包括基因工程、发酵工程等。
二、整体定义
该术语描述将生态学原理与合成生物技术结合的交叉领域,旨在通过人工设计生物系统解决环境问题或实现可持续生产。典型应用包括:
开发可降解污染物的工程微生物;
利用合成生物学优化生物能源生产流程;
设计生态友好的生物制造工艺(如替代化工合成)。
三、技术关联性
其核心逻辑与合成生物学的“设计-构建-测试”范式一致34,但额外强调对生态环境影响的评估与调控。