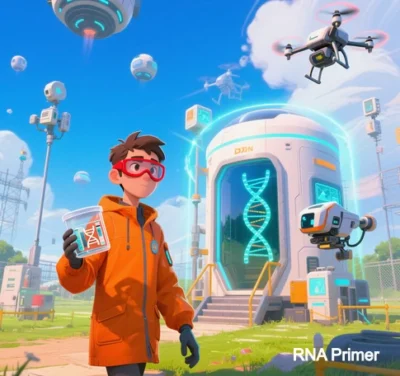
RNA Primers vs. DNA Primers: Core Differences and Applications
RNA primers and DNA primers are two critical nucleotide sequences in molecular biology, serving distinct roles in genetic information transfer in vivo and in vitro. Below is a systematic comparison across five dimensions: composition, function, applications, stability, and molecular mechanisms.
I. Chemical Composition and Structural Differences
Feature | RNA Primer | DNA Primer |
---|---|---|
Nucleotide Type | Ribonucleotides (contain uracil, U) | Deoxyribonucleotides (contain thymine, T) |
Strand Structure | Single-stranded | Double-stranded (forward/reverse primer pair) |
Typical Length | 8–12 nucleotides (short) | 18–24 nucleotides (long) |
3′-OH Group | Provides initiation site for DNA polymerase | Also provides initiation site; retained in product |
Synthesis Method | Biosynthesized by primase | Chemically synthesized or enzymatically prepared |
Key Notes:
- RNA primers contain a 2′-hydroxyl group, making them susceptible to ribonuclease (RNase) degradation.
- DNA primers are more stable due to the absence of the 2′-OH group in deoxyribose.
II. Biological Functions and Mechanisms
1. RNA Primers: The “Molecular Igniter” of DNA Replication
- Core Function: Provide the 3′-OH group required by DNA polymerase III to overcome its inability to initiate de novo synthesis during DNA replication.
- Process:
- Primase recognizes replication origins and synthesizes short RNA primers.
- DNA polymerase III extends primers to form Okazaki fragments.
- DNA polymerase I removes RNA primers via 5’→3′ exonuclease activity and fills gaps with DNA.
- Evolutionary Significance: Supports the “RNA world” hypothesis, reflecting remnants of early genetic systems.
2. DNA Primers: The “Precision Navigator” for In Vitro Amplification
- Core Function: Guide Taq polymerase to target regions via base pairing in PCR.
- Design Principles:
- Specificity: Avoid off-target binding (requires GC content and melting temperature [Tm] optimization).
- Stability: Withstand high-temperature cycling (>95°C denaturation steps).
III. Application Scenarios and Experimental Requirements
Scenario | RNA Primer | DNA Primer |
---|---|---|
In Vivo Processes | DNA replication (prokaryotes/eukaryotes) | Not applicable |
In Vitro Techniques | Rarely used (except in reverse transcription) | PCR, DNA sequencing, gene cloning |
Removal Mechanism | Required (via RNase H or DNA polymerase I) | Retained in the final product |
Temperature Sensitivity | Degrades at high temperatures (limits in vitro use) | Thermostable (compatible with PCR cycling) |
Examples:
- RNA Primer: Initiates viral DNA synthesis in African swine fever virus replication.
- DNA Primer: Specific primers amplify the ORF1ab gene in COVID-19核酸检测.
IV. Stability and Error-Correction Mechanisms
Aspect | RNA Primer | DNA Primer |
---|---|---|
Thermal Stability | Low (2′-OH accelerates hydrolysis) | High (deoxyribose resists heat) |
Enzyme Sensitivity | Degraded by RNases | Requires DNase for degradation |
Proofreading | No intrinsic proofreading (replaced post-use) | High-fidelity enzymes have 3’→5′ exonuclease activity |
Storage Conditions | Short-term at -80°C | Long-term at -20°C |
Experimental Impact: RNA primers are unsuitable for multi-cycle PCR due to instability, while DNA primers are reusable.
V. Synthesis Pathways and Cost Efficiency
Factor | RNA Primer | DNA Primer |
---|---|---|
Synthesis Enzyme | Primase (ATP-dependent) | Chemical synthesis (solid-phase phosphoramidite method) |
Time Cost | Milliseconds (in vivo synthesis) | Hours (commercial synthesis) |
Economic Cost | Low (autonomous in vivo synthesis) | High (commercial synthesis per base) |
Customization Flexibility | Fixed sequence (template-dependent) | Highly customizable (user-defined targets) |
Emerging Trends: CRISPR-Cas9 has been used to engineer primase for specific RNA primer design, though in vitro applications remain limited.
VI. Summary Comparison
Aspect | RNA Primer | DNA Primer |
---|---|---|
Core Role | Temporary initiator for DNA replication | Permanent anchor for in vitro amplification |
Persistence | Transient (replaced post-replication) | Permanent (integrated into final product) |
Application Limits | Restricted to natural in vivo processes | Adaptable to diverse molecular techniques |
Evolutionary Role | Relic of primordial genetic systems | Engineered tool of modern biotechnology |
VII. Future Research Directions
- Synthetic Biology: Develop RNA/DNA hybrid primers to merge stability and functional flexibility.
- Diagnostics: Design multiplex PCR panels with DNA primers for one-step pathogen screening.
- Gene Therapy: Engineer primase to generate therapeutic RNA primers for mutation repair in vivo.
RNA and DNA primers play complementary roles: the former is a “primordial tool” shaped by natural selection, while the latter is a “molecular scalpel” honed by human ingenuity. Understanding their differences is key to optimizing experiments and decoding biological processes.
Data sourced from public references. Contact: chuanchuan810@gmail.com.