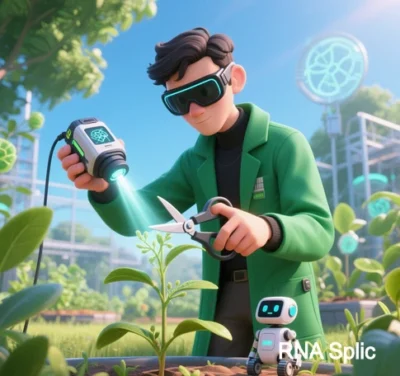
RNA Splicing: The Precision-Driven Link Between Transcription and Translation
RNA splicing is a critical biological process that ensures the accurate conversion of genetic information from pre-mRNA to mature mRNA. By removing non-coding introns and joining exons, splicing bridges transcription and translation, directly impacting protein diversity and cellular function. Below is a structured analysis of its mechanisms, regulatory networks, and implications for health and disease.
I. Core Mechanisms of RNA Splicing
- Spliceosome Machinery:
- Components:
- snRNPs (U1, U2, U4, U5, U6): Recognize splice sites (GU-AG rule) and catalyze intron excision.
- Splicing Factors (e.g., SR proteins, hnRNPs): Enhance or repress splice site selection.
- Process:
- Intron Lariat Formation: U2 snRNP binds branch site adenosine, initiating transesterification.
- Exon Ligation: U5 snRNP aligns exons for ligation via ATP-dependent remodeling.
- Alternative Splicing:
- Mechanisms: Exon skipping, intron retention, alternative 5’/3′ splice sites.
- Functional Impact: Generates >90% human protein diversity from ~20,000 genes (e.g., DSCAM in Drosophila yields 38,016 isoforms).
II. Precision Regulation: Ensuring Fidelity
- Cis-Elements:
- Splice Sites: Canonical GU-AG sequences (99.9% of introns).
- Exonic/Intronic Splicing Enhancers (ESE/ISE): Recruit SR proteins (e.g., SRSF1) to promote splicing.
- Exonic/Intronic Splicing Silencers (ESS/ISS): Bind hnRNPs (e.g., hnRNP A1) to block spliceosome assembly.
- Trans-Factors:
- Kinases (e.g., CLK, SRPK): Phosphorylate SR proteins to modulate splice site selection.
- Epigenetic Regulation: Histone modifications (e.g., H3K36me3) guide splicing factors to nascent RNA.
III. Splicing Errors and Human Diseases
- Cancer:
- Oncogenic Isoforms: BCL-XL (anti-apoptotic) over BCL-XS in lung cancer; aberrant FGFR2 splicing in gastric cancer.
- Mutated Splice Factors: SF3B1 mutations in myelodysplastic syndromes drive mis-splicing of BRD9 and MAP3K7.
- Neurodegenerative Disorders:
- Tauopathies: Mis-splicing of MAPT exon 10 increases 4R-tau, linked to Alzheimer’s and frontotemporal dementia.
- Spinal Muscular Atrophy (SMA): SMN2 exon 7 skipping reduces survival motor neuron protein.
- Metabolic Diseases:
- Familial Dyslipidemia: LDLR exon 4 skipping impairs cholesterol uptake.
IV. Cutting-Edge Technologies and Therapies
- Diagnostics:
- RNA-Seq + Machine Learning: SpliceBERT predicts splicing variants from sequence context (AUC >0.95).
- Nanopore Direct RNA Sequencing: Detects real-time splicing intermediates in single molecules.
- Therapeutic Interventions:
- Antisense Oligonucleotides (ASOs):
- Spinraza (nusinersen): Binds SMN2 ISS-N1 to promote exon 7 inclusion (FDA-approved for SMA).
- Eteplirsen: Skips DMD exon 51 in Duchenne muscular dystrophy.
- CRISPR-Based Splicing Modulation:
- dCas9-SF3B1: Reprograms splice site selection in BRCA1 to restore functional isoforms.
- Prime Editing: Corrects CFTR exon 9 cryptic splice sites in cystic fibrosis.
- Small-Molecule Splicing Modulators:
- H3B-8800: Inhibits SF3B1 to selectively kill spliceosome-mutant cancers (Phase II trials).
V. Future Directions
- Spatiotemporal Splicing Maps: Single-cell and spatial transcriptomics to resolve splicing heterogeneity in tissues.
- AI-Driven Drug Design: Deep learning predicts off-target effects of ASOs and small molecules.
- Synthetic Biology: Engineered spliceosomes for on-demand isoform production in gene therapy.
Conclusion
RNA splicing is not merely a step in gene expression but a precision-controlled hub shaping proteomic complexity and cellular identity. Its dysregulation underpins numerous diseases, yet advances in splicing-targeted therapies (e.g., ASOs, CRISPR) offer transformative potential. Future breakthroughs will hinge on integrating multi-omics data, AI, and synthetic biology to decode and harness splicing’s full regulatory logic.
Data sourced from public references. Contact: chuanchuan810@gmail.com.