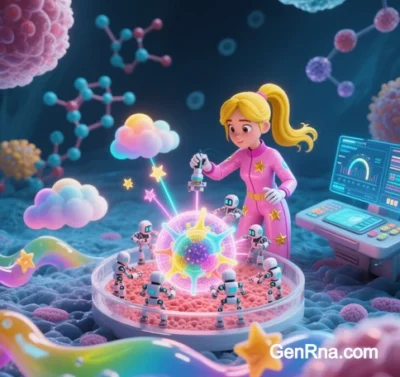
Latest Advances in Synthetic Biology
(2025 Comprehensive Review)
Synthetic biology integrates engineering, computer science, and life sciences to design and construct artificial biological systems or reengineer natural systems for predictable and programmable functions. Here are the core advancements in the field:
I. Design and Construction of Artificial Biological Systems
1. Artificial Genomes and Chromosomal Engineering
- Whole Genome Synthesis: Building on the 2010 synthesis of the Mycoplasma genome (JCVI-syn1.0), technologies now extend to eukaryotes. For example, Chinese researchers engineered Z-fold artificial chromosomes mimicking 30 nm chromatin fibers, revealing links to gene silencing and offering new therapeutic targets.
- Non-Natural Genetic Systems: A team at UC Irvine developed efficient TNA polymerases to synthesize threose nucleic acid (TNA), which is more stable than DNA and ideal for degradation-resistant biosensors and drug delivery.
2. Orthogonal Genetic Systems and Biomolecular Engineering
- Non-Natural Bases and Amino Acids: Expanding the genetic code with unnatural base pairs (e.g., dNaM-dTPT3) and amino acids (e.g., photo-crosslinking groups) enables novel chemical diversity. Orthogonal tRNA/synthetase systems insert fluorescent amino acids into eukaryotic cells for live protein tracking.
- Mirror-Image Life Systems: Chemically synthesized mirror DNA polymerases replicate and transcribe mirror DNA, paving the way for enzyme-resistant drugs and biosecurity materials.
3. Artificial Cells and Synthetic Organisms
- Minimal Cell Factories: Streamlined bacterial genomes (e.g., E. coli MDS42) with simplified metabolic networks produce complex molecules like paclitaxel precursors.
- Multicellular Systems: Engineered bacteria-yeast hybrids use quorum-sensing circuits for light-controlled biofilm formation and metabolite exchange.
II. Reengineering Natural Biological Systems
1. Metabolic Engineering and Cell Factories
- High-Value Products: CRISPR-optimized yeast produces artemisinic acid (malaria drug precursor) and biodegradable PHA plastic at 15x and 20x higher yields, respectively.
- Carbon Capture: Engineered cyanobacteria convert CO₂ to isobutanol (biofuel) via formate dehydrogenase pathways, achieving 85% theoretical efficiency.
2. Genetic Circuits and Smart Regulation
- Therapeutic Applications: CAR-T cells with IF-THEN logic gates activate only in tumor microenvironments (CD19 + hypoxia), reducing off-target toxicity.
- Environmental Sensing: pH-responsive promoters enable gut-targeted anti-inflammatory molecule release in colitis mouse models.
3. Pathogen Engineering and Biosecurity
- Phage Evolution: Synthetic phages target antibiotic-resistant bacteria (e.g., MRSA) with lytic enzymes and antibiotic sensitizers, boosting killing efficiency by 90%.
- Self-Limiting Gene Drives: CRISPR-based systems control malaria mosquito populations, deactivating after 10 generations to mitigate ecological risks.
III. Core Enabling Technologies
1. Gene Editing and Synthesis
- CRISPR-Cas12f Variants: Ultracompact Cas12f (400 amino acids) enables efficient in vivo editing, tested in retinal degeneration mouse models.
- Seamless DNA Assembly: Gibson Assembly and yeast recombination achieve error-free splicing of 100 kb DNA fragments.
2. AI-Driven Biological Design
- AlphaFold3 + Rosetta: Predicts protein-nucleic acid structures and optimizes enzymes (e.g., AI-designed PET hydrolases degrade plastic 30x faster than natural enzymes).
- Automated Platforms: High-throughput systems (e.g., Opentron) test 10,000 promoter-RBS combinations weekly, accelerating pathway optimization.
3. Cell-Free Synthesis
- In Vitro Production: E. coli-based systems synthesize mRNA vaccines and virus-like particles (VLPs) 70% faster than cell culture.
- Modular BioBricks: A library of 50,000 standardized parts enables plug-and-play pathway assembly (e.g., plant terpenoid synthesis in yeast).
IV. Application Domains
Field | Examples | Key Technologies |
---|---|---|
Biomedicine | Personalized neoantigen vaccines, gene-edited CAR-T, senolytic drug screening | Genetic circuits, AI design |
Energy & Environment | Microbial fuel cells, PHA bioplastics, artificial photosynthesis | Metabolic engineering, synthetic genomes |
Agriculture & Food | Nitrogen-fixing bacteria, high-vitamin C tomatoes, cultured meat (serum-free media) | CRISPR editing, cell factories |
Industrial Materials | Spider silk mimics, self-healing biocement, DNA data storage (215 PB/g) | Orthogonal systems, DNA synthesis |
V. Challenges and Future Directions
- Technical Hurdles:
- Complexity: Dynamic regulation of multi-gene circuits requires systems biology models.
- Scalability: Industrial-scale cell factories face metabolic burden and toxicity; robust chassis cells are needed.
- Ethics and Safety:
- Biocontainment: Synthetic organisms require “kill switches” (e.g., temperature-sensitive toxins) to prevent environmental release.
- Regulation: Global frameworks for synthetic biology products are under development, including China’s white paper on risk assessment.
- Interdisciplinary Synergy:
- Bio-Electronic Interfaces: Microbial-based biocomputers using quorum sensing for parallel computation.
- Space Biology: Radiation-resistant “space microbes” for in situ resource production on Mars.
Conclusion
Synthetic biology is transitioning from proof-of-concept to industrial deployment. 2025 milestones include the first AI-designed drug entering trials, cost-competitive PHA bioplastics, and the launch of carbon-neutral biofoundries. Over the next decade, the integration of quantum biocomputing and single-cell omics will enable full-scale programming of life systems—from molecules to ecosystems.
Data sourced from public references. For collaborations, contact: chuanchuan810@gmail.com.